Association between urine microscopy and severe acute kidney injury in critically ill patients following non-cardiac surgery: a prospective cohort study
Introduction
Acute kidney injury (AKI) is an important complication after non-cardiac surgery which has a high incidence (1,2), especially in critically ill surgical patients with rate up to nearly 40% (3). Several underlying susceptibilities, procedures, or exposures have been recognized as risk factors of AKI during the perioperative period (4). More and more evidence has verified the deleterious impact of AKI, especially severe AKI on both short and long term outcomes of surgical patients. Compared to patients with non or mild AKI, patients with severe AKI were associated with increased mortality and higher risk of progressing to chronic kidney disease (CKD) and cardiovascular diseases. Severe AKI patients also had longer length of hospital stay, increased healthcare costs and an increased likelihood of discharge to a health care facility (2,3,5-8). Unfortunately, so far no specific treatment for severe AKI has been established (9), strongly suggesting the necessity of early recognizing patients who will develop severe AKI.
Urine microscopy is an easily accessible and economical test that is widely used for clinical assessment to predict or diagnose kidney disease and monitor the course of disease or response to therapy in those with known kidney disease (10). Accumulated studies have demonstrated the evaluation of urine sediment was especially helpful in early detection of AKI (11,12), differentiating renal AKI from pre-renal AKI (13) and predicting the progression of AKI (14,15). For different patient populations, urine microscopy showed a very high specificity in the diagnosis of AKI in patients from emergency department (12) or undergoing open cardiac surgery (16).
However, limited data focused on critically ill patients following non-cardiac surgery, of which AKI presented a distinct pathophysiology from other types of AKI (4). We hypothesized that critically ill patients with severe AKI after non-cardiac surgery would have different patterns of renal tubular cells and/or casts in the urine sediment and that these would provide additional diagnostic information. Therefore, the purpose of this study was to investigate the association between urine microscopy and severe AKI in critically ill patients after non-cardiac surgery. We present the following article in accordance with the STROBE reporting checklist (available at https://apm.amegroups.com/article/view/10.21037/apm-21-3085/rc).
Methods
Ethics and consent
This was a prospective cohort study registered at ClinicalTrials.gov (NCT03880110). The study was conducted in accordance with the Declaration of Helsinki (as revised in 2013). The study was approved by the Clinical Research Ethics Committee of Peking University First Hospital on April 10, 2019 (No.: 2019-075) and informed consent was taken from all the patients.
Patients
This study was performed from May 20, 2019 to November 24, 2020 in a teaching hospital affiliated with a university. Patients with at least one of the following conditions would admit to SICU after surgery: (I) preoperative comorbidities, including hypertension, diabetes mellitus, coronary heart disease, congestive heart failure, cerebrovascular disease, CKD and so on; (II) major surgery, defined as surgery duration ≥2 h; (III) ongoing organ dysfunction, defined as the sequential organ failure assessment (SOFA) score ≥2 from one single organ system. In this study, adult patients undergoing non-cardiac surgery and then immediately admitted to surgical intensive care unit (SICU) were consecutively enrolled. Patients with any of the following criteria were excluded: (I) CKD stage 5 or requiring long-term dialysis; (II) undergoing kidney-related surgery; (III) AKI events before admission to SICU; (IV) without Foley catheter placement; (V) written informed consent not obtained.
Urine microscopy
Fresh urine samples were collected from patients’ Foley catheters which were placed at the beginning of anesthesia, and then prepared and examined by specially assigned and experienced investigators within 1 hour. Urine samples were centrifuged (Baiyang, 600C) at 400 g for 5 min to maximize yield and concentrated 50-fold by resuspending the deposit. By using an Olympus BX43 phase contrast microscope, urine sediment was evaluated with ×10, ×20 and ×40 magnification objectives and a ×10 magnification eyepiece. A single drop of urine sediment was placed on S-Y Double Grid Counting Slide 10. After low power fields (LPFs) scanning, high power fields (HPFs) were used to further differentiate epithelial cells, renal tubular epithelial cells (RTEC) and granular casts, which were then counted and averaged.
There is a strict protocol for quality control of urine microscopy, including inter-observer variability. Specifically, the department of clinical laboratory will conduct inter-observer comparison every 6 months. In which, 5 urine sediment samples with at least 3 abnormal are prepared, and then casts and cells are examined and averaged under at least 20 LPFs and 10 HPFs, respectively. Taking the results from specialist in urine microscopy as reference, the following criteria are adopted. When casts are ≤10/LPF or >10/LPF, the difference of results should be <3/LPF or <5/LPF, respectively; when cells are ≤10/HPF, between 10 and 100/HPF or >100/HPF, the difference of results should be <3/HPF, <10/HPF or <20/HPF, respectively. Furthermore, the urine microscopy of our clinical laboratory is also qualified by external quality assessment from National Center for Clinical Laboratories twice a year and International Organization for Standardization 15189 reassessment every two years.
Results of urine sediment were graded by urine microscopy score (UMS) outlined in Table 1 (16) and elevated UMS was defined as ≥1.
Table 1
Score [renal tubular epithelial cells per HPF] | Score [granular cast per HPF] | |||
---|---|---|---|---|
0 [0] | 1 [1] | 2 [2–4] | 3 [≥5] | |
0 [0] | 0 | 1 | 2 | 3 |
1 [1] | 1 | 2 | 3 | 4 |
2 [2–4] | 2 | 3 | 4 | 5 |
3 [≥5] | 3 | 4 | 5 | 6 |
HPF, high power field.
Data collection and study outcomes
Patients’ data were prospectively collected during their hospital stay by one special researcher. Follow-up visit was performed according to a prespecified protocol from patients’ enrollment until their discharge, death or 30 days after surgery, whichever came first. Preoperative data included demographic characteristics, body mass index (BMI), medical history, American Society of Anesthesiology (ASA) physical status classification, nephrotoxin exposure and clinical laboratory data; while intraoperative data contained information of surgery and anesthesia (type, location and duration), estimated blood loss, urine output, and management such as vasopressors infusion, blood products transfusion and fluid balance; for postoperative data, urine microscopy immediately, 6 and 12 hours after SICU admission were collected. Non-renal SOFA score and acute physiology and chronic health evaluation II (APACHE II) score within 24 hours of SICU admission were assessed for the severity of illness.
The primary outcome was severe AKI development, defined as AKI stage 2 or 3, within 7 days after surgery. Postoperative AKI and its severity was defined according to Kidney Disease Improving Global Outcomes (KDIGO) criteria using the maximal change in serum creatinine compared with the preoperative baseline values and urine output (17). Serum creatinine was examined at admission to SICU and daily at 6 am within 7 days after surgery, while urine output was closely monitored during the SICU stay.
The secondary outcomes were the postoperative use of mechanical ventilation (MV) and its duration, vasopressors infusion ≥24 hours, length of SICU and postoperative hospital stay, number of postoperative complications other than AKI (Table S1), and in-hospital mortality.
Relevant data underlying the main results is available at https://cdn.amegroups.cn/static/public/apm-21-3085-01.xlsx.
Statistical analysis
Perioperative data were firstly compared among patients without AKI, with mild AKI and with severe AKI, respectively. Then these data were further compared between patients with and without severe AKI. Quantitative variables with normal distribution were compared by independent samples t-test or one-way ANOVA; while Mann-Whitney U or Kruskal-Wallis test was used for non-normal distribution. Qualitative variables were compared by Chi-squared test or Fisher’s exact test. Time-to-event data were analyzed with Kaplan-Meier survival analysis, with difference between groups compared by Log- rank test. After testing for collinearity, perioperative variables with P<0.10 in the univariate analyses or considered clinically important for severe AKI were included in multivariate logistic regression model (backward LR method) to adjust the association between UMS and severe AKI. Two-sided P values <0.05 were regarded as statistically significant. The SPSS v21.0 software package was used for statistical processing (SPSS Inc., Chicago, IL, USA).
Results
A total of 1,105 patients were admitted to SICU during the study period. After screening for inclusion and exclusion criteria, 661 patients were finally recruited (Figure 1). Among the enrolled patients, the incidence of severe AKI within 7 days after surgery was 22.2% (147 patients) with AKI stage 2 and 3 accounting for 20.5% (136 patients) and 1.7% (11 patients), respectively. The median time to AKI and severe AKI diagnosis after SICU admission was 9.3 (6.0, 16.2) and 14.3 (12.0, 21.6) hours. The actual median sampling time for immediately, 6 and 12 hours of urine microscopy was 0.8 (0.0, 0.3), 6.0 (6.0, 6.1) and 12.0 (12.0, 12.1) hours after SICU admission, respectively. Baseline and perioperative data were listed in Table 2 and Table 3. For preoperative variables, age, BMI, history of CKD, hemoglobin and albumin, baseline serum creatinine and estimated glomerular filtration rate (eGFR), use of angiotensin converting enzyme inhibitor (ACEI) or angiotensin receptor blocker (ARB) and smoking habit showed significant difference among groups, while for intra and postoperative variables, intraoperative urine output, postoperative elevated UMS 6 and 12 hours after admission, non-renal SOFA score and APACHE II score after 24 hours of SICU admission were statistically different.
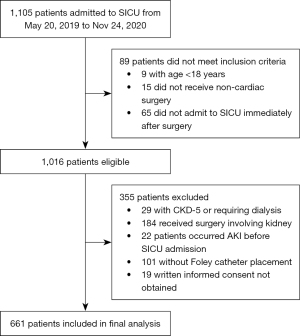
Table 2
Total (n=661) | Without AKI (n=361) | With mild AKI (n=153) | With severe AKI (n=147) | P value | |
---|---|---|---|---|---|
Age (y) | 69±15 | 66±15 | 71±14 | 73±14 | <0.001 |
Male sex | 402 (60.8%) | 229 (63.4%) | 81 (52.9%) | 92 (62.6%) | 0.074 |
BMI (kg/m2) | 24.0±4.0 | 23.5±3.8 | 24.3±4.2 | 24.9±4.3 | 0.001 |
Preoperative comorbidities | |||||
Diabetes mellitus | 187 (28.3%) | 102 (28.3%) | 40 (26.1%) | 45 (30.6%) | 0.691 |
Hypertension | 403 (61.0%) | 207 (57.3%) | 207 (64.7%) | 97 (66.0%) | 0.108 |
Coronary heart disease | 223 (33.7%) | 114 (31.6%) | 51 (33.3%) | 58 (39.5%) | 0.233 |
Congestive heart failure | 86 (13.0%) | 39 (10.8%) | 24 (15.7%) | 23 (15.6%) | 0.180 |
Cerebrovascular disease | 122 (18.5%) | 59 (16.3%) | 30 (19.6%) | 33 (22.4%) | 0.251 |
Chronic kidney disease | 76 (11.5%) | 29 (8.0%) | 25 (16.3%) | 22 (15.0%) | 0.009 |
ASA classification | 0.114 | ||||
I–II | 113 (17.1%) | 71 (19.7%) | 24 (15.7%) | 18 (12.2%) | |
III–IV | 548 (82.9%) | 290 (80.3%) | 129 (84.3%) | 129 (87.8%) | |
Preoperative Hb (g/L)a | 124±22 | 126±21 | 122±22 | 121±22 | 0.014 |
Preoperative albumin (g/L)a | 38.8±5.2 | 39.6±5.1 | 38.2±5.5 | 37.4±5.0 | <0.001 |
Baseline serum Cr (μmol/L)b | 81.1±31.0 | 77.8±24.8 | 86.1±41.0 | 84.0±31.8 | 0.009 |
Baseline eGFR (mL/min/1.73 m2)c | 80.2±21.7 | 83.9±20.3 | 75.7±22.5 | 76.0±22.5 | <0.001 |
Radiocontrast exposured | 56 (8.5%) | 32 (8.9%) | 11 (7.2%) | 13 (8.8%) | 0.810 |
On ACEI/ARB | 104 (15.7%) | 42 (11.6%) | 31 (20.3%) | 31 (21.1%) | 0.006 |
Smoking habite | 213 (32.2%) | 130 (36.0%) | 31 (20.3%) | 52 (35.4%) | 0.001 |
Data are presented as mean ± SD or number of patients (percentage) and compared by one-way ANOVA, Kruskal-Wallis test or chi-squared test/Fisher’s exact test respectively. a, measured within 3 days before surgery. b, determined by the minimal value of serum creatinine measured within 3 months before admission and in hospital before surgery; if neither value was available, the modification of diet in renal disease formula was adopted to estimate the baseline serum creatinine according to the Kidney Disease Improving Global Outcomes guideline. c, estimated by the modification of diet in renal disease formula. d, including patients who had radiocontrast exposure within 2 days before surgery. e, smoking for more than 10 cigarettes per day for more than 1 year, including current or past smokers. AKI, acute kidney injury; BMI, body mass index; ASA, American society of anesthesiologists; Hb, hemoglobin; Cr, creatinine; eGFR, estimated glomerular filtration rate; ACEI, angiotensin converting enzyme inhibitor; ARB, angiotensin receptor blocker; ANOVA, analysis of variance.
Table 3
Total (n=661) | Without AKI (n=361) | With mild AKI (n=153) | With severe AKI (n=147) | P value | |
---|---|---|---|---|---|
Duration of anesthesia (min) | 292±141 | 293±135 | 297±156 | 282±140 | 0.642 |
Duration of surgery (min) | 212±134 | 214±128 | 214±149 | 206±134 | 0.824 |
Emergency surgery | 39 (5.9%) | 20 (5.5%) | 11 (7.2%) | 8 (5.4%) | 0.742 |
Open surgerya | 243 (46.2%) | 118 (42.1%) | 63 (51.6%) | 62 (50.0%) | 0.134 |
Type of surgery | 0.193 | ||||
Abdominal and pelvic surgery | 447 (67.6%) | 240 (66.5%) | 99 (64.7%) | 108 (73.5%) | |
Thoracic surgery | 67 (10.1%) | 34 (9.4%) | 20 (13.1%) | 13 (8.8%) | |
Neurosurgery | 33 (5.0%) | 25 (6.9%) | 5 (3.3%) | 3 (2.0%) | |
Orthopedic surgery | 98 (14.8%) | 52 (14.4%) | 27 (17.6%) | 19 (12.9%) | |
Othersb | 16 (2.4%) | 10 (2.8%) | 2 (1.3%) | 4 (2.7%) | |
Estimated blood loss (mL) | 100 [50, 300] | 100 [50, 300] | 100 [50, 400] | 100 [50, 300] | 0.746 |
Urine output (mL/kg/h) | 1.5 (0.9, 2.6) | 1.7 (1.0, 2.9) | 1.4 (0.8, 2.1) | 1.2 (0.7, 1.9) | <0.001 |
Intraoperative management | |||||
Vasopressors infusioc | 334 (50.5%) | 181 (50.1%) | 75 (49.0%) | 78 (53.1%) | 0.764 |
Blood products transfusiond | 146 (22.1%) | 77 (21.3%) | 43 (28.1%) | 26 (17.7%) | 0.082 |
Fluid balance (mL/kg/h) | 6.5±4.2 | 6.8±4.7 | 6.6±3.8 | 5.8±3.0 | 0.058 |
Postoperative variables | |||||
Elevated UMS at admissione | 56 (8.5%) | 24 (6.6%) | 13 (8.5%) | 19 (12.9%) | 0.070 |
Elevated UMS 6 hours after admissione | 55 (8.3%) | 20 (5.5%) | 12 (7.8%) | 23 (15.6%) | 0.001 |
Elevated UMS 12 hours after admissione | 61 (9.3%) | 14 (3.9%) | 18 (11.8%) | 29 (19.7%) | <0.001 |
Non-renal SOFA scoref | 3 (2, 4) | 2 (2, 4) | 3 (2, 4) | 3 (2, 5) | <0.001 |
APACHE II scoref | 12±4 | 12±4 | 12±4 | 13±5 | <0.001 |
D0 Fluid balance (mL/kg) | 32.0 (19.8, 46.5) | 29.9 (17.4, 43.3) | 34.3 (21.5, 49.7) | 33.5 (22.7, 48.9) | 0.012 |
D0 Urine output (mL/kg) | 20.4 (12.7, 31.7) | 25.7 (15.9, 36.6) | 18.8 (12.1, 26.6) | 12.6 (8.3, 18.4) | <0.001 |
Data are presented as mean ± SD, median (interquartile range), or number of patients (percentage) and compared by one-way ANOVA, Kruskal-Wallis test or chi-squared test/Fisher’s exact test respectively. a, open or laparoscopic surgery referred to 526 patients. b, including peripheral vascular surgery, ear, nose and throat surgery and thyroid or breast surgery. c, including use of phenylephrine, norepinephrine, epinephrine, and dopamine. d, including packed red blood cell, plasma, and platelet. e, defined as UMS ≥1. f, calculated after 24 hours of SICU admission. AKI, acute kidney injury; UMS, urine microscopy score; SOFA, sequential organ failure assessment score; APACHE, acute physiology and chronic health evaluation; D0, the day of surgery; ANOVA, analysis of variance; SICU, surgical intensive care unit.
In contrast to patients without AKI and with mild AKI (Tables S2,S3), patients with severe AKI had higher age and BMI, lower baseline eGFR and decreased level of preoperative hemoglobin and albumin. More patients with severe AKI used ACEI or ARB preoperatively. In addition, less intraoperative urine output and fluid balance were seen in severe AKI patients, who also had higher non-renal SOFA score and APACHE II score.
Furthermore, as shown in Table S3, more patients with severe AKI had elevated UMS immediately, 6 and 12 hours after SICU admission than patients without severe AKI (12.9% vs. 7.2%, P=0.028; 15.6% vs. 6.2%, P<0.001; 19.7% vs. 6.3%, P<0.001, respectively). In multivariate logistic regression model, elevated UMS 6 and 12 hours after SICU admission were independently associated with postoperative severe AKI (OR 2.200, 95% CI: 1.182–4.095, P=0.013 and OR 2.949, 95% CI: 1.657–5.248, P<0.001, respectively) after adjusting for age, BMI, history of coronary heart disease and CKD, ASA classification, preoperative hemoglobin and albumin, baseline eGFR, use of ACEI or ARB, intraoperative urine output and fluid balance and non-renal SOFA score after 24 hours of SICU admission (Table 4). In addition, as shown in Figure 2, UMS began to elevate 8 hours earlier than the diagnosis of severe AKI.
Table 4
Univariate logistic model | Multivariate logistic modela | ||||
---|---|---|---|---|---|
OR (95% CI) | P value | OR (95% CI) | P value | ||
Elevated UMS at admission | 1.914 (1.064–3.440) | 0.030 | – | – | |
Elevated UMS 6 hours after admission | 2.794 (1.578–4.945) | <0.001 | 2.200 (1.182–4.095) | 0.013 | |
Elevated UMS 12 hours after admission | 3.686 (2.145–6.335) | <0.001 | 2.949 (1.657–5.248) | <0.001 |
a, factors with P values <0.10 in univariate analyses or considered clinically important were included in the multivariate logistic regression model with backward LR method for adjustment. These included age, body mass index, history of coronary heart disease and chronic kidney disease, ASA classification, preoperative hemoglobin and albumin, baseline eGFR, use of ACEI or ARB, intraoperative urine output and fluid balance and non-renal SOFA score after 24 hours of SICU admission. CI, confidence interval; OR, odds ratio; UMS, urine microscopy score; ASA, American Society of Anesthesiologists; eGFR, estimated glomerular filtration rate; ACEI, angiotensin converting enzyme inhibitor; ARB, angiotensin receptor blocker.
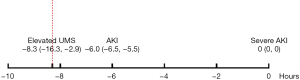
Moreover, taking UMS 0 as reference, higher UMS 6 hours after SICU admission demonstrated well correlation with greater risk of severe AKI with OR 3.887 (95% CI: 1.430–10.563) for UMS ≥3 and OR 2.429 (95% CI: 1.237–4.770) for UMS =1–2. These relationships remained even after adjusting for the above factors with OR 3.097 (95% CI: 1.050–9.139) for UMS ≥3 and OR 1.952 (95% CI: 0.942–4.044) for UMS =1–2 (Figure 3). The specificity of UMS ≥3 and ≥1 for severe AKI were 98.4% (95% CI: 97.3–99.5%) and 93.8% (95% CI: 91.7–95.9%), while the sensitivity were 5.4% (95% CI: 1.7–9.1%) and 15.6% (95% CI: 9.7–21.5%), respectively. The positive predictive values of UMS ≥3 and ≥1 for severe AKI were 50.0% (95% CI: 25.5–74.5%) and 41.8% (95% CI: 28.8–54.8%), and the negative predictive values were 78.4% (95% CI: 75.2–81.6%) and 79.5% (95% CI: 76.3–82.7%), respectively.
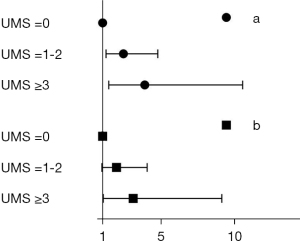
To improve the usefulness of UMS in clinical situation, we further analyzed risk factors related to the development of postoperative severe AKI (Table S4) and the results showed that age (y) [OR 1.026 (95% CI: 1.010–1.042)], BMI (kg/m2) [OR 1.082 (95% CI: 1.028–1.140)], preoperative albumin (g/L) [OR 0.956 (95% CI: 0.919–0.994)] and intraoperative urine output (mL/kg/h) [OR 0.818 (95% CI: 0.688–0.972)] were independently associated with severe AKI.
In addition, patients with higher UMS (≥3, 1–2 and 0) after 6 hours of admission had significantly more postoperative complications [1 (0, 2), 0 (0, 1) and 0 (0, 0), P=0.018] (Table 5, Table S1) and longer SICU stay [3.0 (1.1, 4.9), 2.0 (1.3, 2.7) and 1.0 (0.9, 1.1), P=0.015]; and they also showed a trend toward other adverse postoperative outcomes, such as longer duration of MV and vasopressors infusion; more days of postoperative hospital stay and increased in-hospital mortality (Table 5).
Table 5
UMSa =0 (n=606) | UMSa =1–2 (n=39) | UMSa ≥3 (n=16) | P value | |
---|---|---|---|---|
MV | 301 (49.7%) | 24 (61.5%) | 10 (62.5%) | 0.225 |
Duration of MV (h)b | 6.0 (5.3, 6.8) | 9.0 (5.4, 12.6) | 10.0 (0.0, 22.4) | 0.354 |
Vasopressors infusion ≥24 hoursc | 44 (7.3%) | 5 (12.8%) | 3 (18.8%) | 0.120 |
Number of postoperative complicationsd | 0 (0, 0) | 0 (0, 1) | 1 (0, 2) | 0.018 |
Length of SICU stay (d)e | 1.0 (0.9, 1.1) | 2.0 (1.3, 2.7) | 3.0 (1.1, 4.9) | 0.015 |
Postoperative hospital stay (d)e | 9.0 (8.5, 9.5) | 11.0 (8.4, 13.6) | 12.0 (7.3, 16.7) | 0.257 |
In-hospital mortality | 10 (1.7%) | 0 (0.0%) | 1 (6.3%) | 0.257 |
Data are presented as number of patients (percentage) or median (interquartile range), unless otherwise indicated. a, urine microscopy scores 6 hours after SICU admission. b, results of patients requiring postoperative mechanical ventilation. Data were analyzed by Kaplan-Meier analysis and compared by Log-rank test; results are presented as median (95% confidence interval). c, including use of phenylephrine, norepinephrine, epinephrine, and dopamine. d, including pulmonary infection, pleural effusion, atelectasis, respiratory failure, acute myocardial infarction, new-onset arrhythmia, hemodynamic insufficiency, stroke, venous thromboembolism, ileus, intra-abdominal abscess, wound infection, urinary tract infection, sepsis, surgical bleeding, digestive tract bleeding, acute liver injury, disseminated intravascular coagulation, anastomotic leakage, and wound dehiscence (Table S1). e, data were analyzed by Kaplan-Meier analysis and compared by Log-rank test; results are presented as median (95% confidence interval). UMS, urine microscopy score; MV, mechanical ventilation; SICU, surgical intensive care unit.
Discussion
Results of this prospective study demonstrated that urine microscopy 6 hours after SICU admission was independently associated with severe AKI occurrence in critically ill patients following non-cardiac surgery with higher UMS related to greater risk. Elevated UMS had a high specificity and negative predictive value, but a relatively low sensitivity and positive predictive value for severe AKI detection. Furthermore, patients with increased UMS tended to have worse outcomes. Considering the lack of data on the relationship between urine microscopy and AKI after non-cardiac surgery, our study provided valuable information and strengthened evidence on this field.
In our study, the incidence of severe AKI (defined as AKI stage ≥2) after surgery was 22.2%, while in the study conducted by Park and colleagues (18), the incidence of critical AKI (defined as AKI stage ≥2 and AKI that consequently led to post-AKI death or dialysis) was 1.1% in the discovery cohort and 1.5% in the validation cohort, respectively. We thought there were two possible reasons. Firstly, compared to Park’s study which included patients in general ward, our study mainly focused on critically ill patients following non-cardiac surgery who had more complex and severe perioperative conditions. Secondly, in our study, postoperative AKI and its severity was defined according to both serum creatinine and urine output criteria, while Park’s study only used serum creatinine criteria which might underestimate the incidence of severe AKI.
Several studies have been undertaken to elucidate the relationship between urine microscopy and early detection of AKI. In 1979, the research of Schentag et al. (11). demonstrated that urinary casts were significantly higher in nephrotoxic AKI, defined as increase in serum creatinine ≥0.5 mg/dL within 5 days after aminoglycoside treatment, and increased as early as 9 days before changes of serum creatinine in patients from intensive care unit. Schinstock and colleagues (12) conducted a study focused on emergency patients and found that the overall agreement of presence of any RTECs or RTEC/granular casts and severe AKI was 85.4% (95% CI: 81.4–88.7%). In a study on patients following open cardiac surgery, urine sediment were significantly higher in AKI patients and appeared as early as just 2 hours after termination of cardiopulmonary bypass (CPB) (19). Our data showed a higher rate of elevated UMS in severe AKI patients even just immediately after surgery and the independent association of UMS 6 hours after SICU admission with severe AKI. UMS elevated as early as 8 hours before diagnosis of severe AKI. All of which would reinforce the previous findings and suggested that early elevated UMS in critically ill patients after non-cardiac surgery was independently associated with severe AKI. Furthermore, higher UMS was related to greater risk.
In accordance with the study on emergency patients (12), in which abnormal urine microscopy had a specificity of 89.9% (95% CI: 86.2–92.7%) and sensitivity of 29.6% (95% CI: 15.9–48.5%) for severe AKI, our results showed the specificity and sensitivity for severe AKI were 93.8% (95% CI: 91.7–95.9%) and 15.6% (95% CI: 9.7–21.5%), respectively. However, urine microscopy 12 hours after CPB was found to be highly sensitive and specific for cardiac surgery related AKI with area under the curve of 0.888 (95% CI: 0.746–1.029) (19). The possible explanation might be that patients undergoing cardiac surgery, especially with CPB, were exposed to a number of risk factors and then several mechanisms, such as ischemia-reperfusion injury, inflammation, oxidative stress, hemolysis and nephrotoxins, combined together to result in more severe renal tubular injury (20) which likely had positive manifestations of RTECs or RTEC/granular casts in urine sediment.
Urine output was a key diagnostic criterion of AKI, but its reliability in perioperative patients was getting controversial because there was a physiologic reduction in urine output as a result from hypovolemia, anesthesia and release of aldosterone and vasopressin in response to stress (21). In addition, elevations in serum creatinine might also not always represent renal injury which was commonly contributed to hemodynamic reduction in renal blood flow during perioperative period (22). As shown in Table 3, patients with severe AKI had significantly reduced intraoperative and D0 (the day of surgery) urine output. Furthermore, although severe AKI patients had more positive D0 fluid balance, there was a trend that they received less fluid balance during surgery. Therefore, pre-renal factor might partly contribute to the development of severe AKI. Differentiating structural AKI from those functional AKI was rather important for risk stratification and subsequent management in patients undergoing non-cardiac surgery. Searching for cell types and casts by urine microscopy examination was very helpful in differential diagnosis of the AKI causes. A purely pre-renal AKI often resulted in urinary sediment that was bland or characterized by hyaline casts, while the presence of RTECs or RTEC/granular casts usually indicated tubular structural injury (10).
In addition, due to higher UMS suggesting more severe renal tubular injury, urine microscopy could also be utilized as a test of predicting short-term prognosis. In our study, patients with early elevated UMS had significantly more postoperative complications and longer SICU stay; and they also showed a trend toward other adverse postoperative outcomes. Several studys have also demonstrated that, in patients with AKI, UMS was positively associated with increase in AKI stage, renal replacement therapy, renal non-recovery and even death during hospitalization (14-16,23).
Our study demonstrated that elevated UMS 6 and 12 hours after SICU admission was independently associated with postoperative severe AKI. Considering the limited time window for renal protection, we thought the time point for measuring UMS might be 6 hours after surgery. Elevated UMS showed a high specificity (93.8%) and negative predictive value (79.5%), while its sensitivity (15.6%) and positive predictive value (41.8%) were low. On one hand, urine microscopy early after non-cardiac surgery could be used as a measurement assisting severe AKI exclusion in patients with risk factors, such as higher age and BMI, preoperative hypoalbuminemia and intraoperative oliguria (Table S4). On the other hand, urine microscopy could be combined with other more sensitive novel injury biomarkers to improve early AKI detection, such as neutrophil gelatinase-associated lipocalin and kidney injury molecule-1 (12,19,24). Furthermore, as an easily accessible and economical examination, urine microscopy might play a more important role in resource limited regions. To sum up, the observations in our study would further assert the complementary value of inclusion of urine microscopy in early risk stratification and diagnosis of severe AKI in critically ill patients following non-cardiac surgery.
There were some limitations in our study. Firstly, postoperative urine output was closely monitored during SICU stay but not when patients transferred back to general ward within 7 days after surgery which might underestimate the incidence and severity of AKI. However, the majority of AKI occurred during the early days of ICU admission (25), thus the possible underestimation was minimized. Secondly, previous study on patients after open cardiac surgery (19) showed UMS began to increase just 2 hours after CPB and nearly achieved peak 12 hours after CPB in AKI patients. Therefore, in this study postoperative urine microscopy was examined immediately, 6 and 12 hours after SICU admission. However, the optimal time point of urine microscopy to independently predict the association with severe AKI still need to be explored. Because of the limited time window leaving for postoperative AKI prevention, our study still provided useful information for future study design. Finally, as a single center study, the generalizability of our results might be limited.
Conclusions
Early urine microscopy was independently associated with severe AKI in critically ill patients following non-cardiac surgery with higher UMS related to greater risk. Urine microscopy was suggested in early risk stratification and diagnosis of severe AKI.
Acknowledgments
The authors gratefully acknowledge Dr. Xi-Zi Zheng (Department of Nephrology, Peking University First Hospital, Beijing, China) and Dr. Mei-Ling Wang (Clinical Laboratory, Peking University First Hospital, Beijing, China) for their suggestions of study design and results interpretation.
Funding: This work was supported by the Interdisciplinary Clinical Research Program of Peking University First Hospital (2018CR32 to SLL). The study sponsor had no role in study design, data acquisition, analysis, interpretation of results, or in the writing of the report.
Footnote
Reporting Checklist: The authors have completed the STROBE reporting checklist. Available at https://apm.amegroups.com/article/view/10.21037/apm-21-3085/rc
Data Sharing Statement: Available at https://apm.amegroups.com/article/view/10.21037/apm-21-3085/dss
Peer Review File: Available at https://apm.amegroups.com/article/view/10.21037/apm-21-3085/prf
Conflicts of Interest: All authors have completed the ICMJE uniform disclosure form (available at https://apm.amegroups.com/article/view/10.21037/apm-21-3085/coif). SLL reports that she received funding of the Interdisciplinary Clinical Research Program of Peking University First Hospital, Beijing, China. The other authors have no conflicts of interest to declare.
Ethical Statement: The authors are accountable for all aspects of the work in ensuring that questions related to the accuracy or integrity of any part of the work are appropriately investigated and resolved. The study was conducted in accordance with the Declaration of Helsinki (as revised in 2013). The study was approved by the Clinical Research Ethics Committee of Peking University First Hospital on April 10, 2019 (No.: 2019-075) and informed consent was taken from all the patients.
Open Access Statement: This is an Open Access article distributed in accordance with the Creative Commons Attribution-NonCommercial-NoDerivs 4.0 International License (CC BY-NC-ND 4.0), which permits the non-commercial replication and distribution of the article with the strict proviso that no changes or edits are made and the original work is properly cited (including links to both the formal publication through the relevant DOI and the license). See: https://creativecommons.org/licenses/by-nc-nd/4.0/.
References
- Bell S, Davey P, Nathwani D, et al. Risk of AKI with gentamicin as surgical prophylaxis. J Am Soc Nephrol 2014;25:2625-32. [Crossref] [PubMed]
- Grams ME, Sang Y, Coresh J, et al. Acute Kidney Injury After Major Surgery: A Retrospective Analysis of Veterans Health Administration Data. Am J Kidney Dis 2016;67:872-80. [Crossref] [PubMed]
- Bihorac A, Yavas S, Subbiah S, et al. Long-term risk of mortality and acute kidney injury during hospitalization after major surgery. Ann Surg 2009;249:851-8. [Crossref] [PubMed]
- McKinlay J, Tyson E, Forni LG. Renal complications of anaesthesia. Anaesthesia 2018;73:85-94. [Crossref] [PubMed]
- Noble RA, Lucas BJ, Selby NM. Long-Term Outcomes in Patients with Acute Kidney Injury. Clin J Am Soc Nephrol 2020;15:423-9. [Crossref] [PubMed]
- Chertow GM, Burdick E, Honour M, et al. Acute kidney injury, mortality, length of stay, and costs in hospitalized patients. J Am Soc Nephrol 2005;16:3365-70. [Crossref] [PubMed]
- O'Connor ME, Kirwan CJ, Pearse RM, et al. Incidence and associations of acute kidney injury after major abdominal surgery. Intensive Care Med 2016;42:521-30. [Crossref] [PubMed]
- Long TE, Helgason D, Helgadottir S, et al. Acute Kidney Injury After Abdominal Surgery: Incidence, Risk Factors, and Outcome. Anesth Analg 2016;122:1912-20. [Crossref] [PubMed]
- Joannidis M, Druml W, Forni LG, et al. Prevention of acute kidney injury and protection of renal function in the intensive care unit: update 2017: Expert opinion of the Working Group on Prevention, AKI section, European Society of Intensive Care Medicine. Intensive Care Med 2017;43:730-49. [Crossref] [PubMed]
- Cavanaugh C, Perazella MA. Urine Sediment Examination in the Diagnosis and Management of Kidney Disease: Core Curriculum 2019. Am J Kidney Dis 2019;73:258-72. [Crossref] [PubMed]
- Schentag JJ, Gengo FM, Plaut ME, et al. Urinary casts as an indicator of renal tubular damage in patients receiving aminoglycosides. Antimicrob Agents Chemother 1979;16:468-74. [Crossref] [PubMed]
- Schinstock CA, Semret MH, Wagner SJ, et al. Urinalysis is more specific and urinary neutrophil gelatinase-associated lipocalin is more sensitive for early detection of acute kidney injury. Nephrol Dial Transplant 2013;28:1175-85. [Crossref] [PubMed]
- Perazella MA, Coca SG, Kanbay M, et al. Diagnostic value of urine microscopy for differential diagnosis of acute kidney injury in hospitalized patients. Clin J Am Soc Nephrol 2008;3:1615-9. [Crossref] [PubMed]
- Perazella MA, Coca SG, Hall IE, et al. Urine microscopy is associated with severity and worsening of acute kidney injury in hospitalized patients. Clin J Am Soc Nephrol 2010;5:402-8. [Crossref] [PubMed]
- Hall IE, Coca SG, Perazella MA, et al. Risk of poor outcomes with novel and traditional biomarkers at clinical AKI diagnosis. Clin J Am Soc Nephrol 2011;6:2740-9. [Crossref] [PubMed]
- Bagshaw SM, Haase M, Haase-Fielitz A, et al. A prospective evaluation of urine microscopy in septic and non-septic acute kidney injury. Nephrol Dial Transplant 2012;27:582-8. [Crossref] [PubMed]
- Kellum JA, Lameire N, Aspelin P, et al. KDIGO clinical practice guideline for acute kidney injury 2012. Kidney Int 2012;1-138.
- Park S, Cho H, Park S, et al. Simple Postoperative AKI Risk (SPARK) Classification before Noncardiac Surgery: A Prediction Index Development Study with External Validation. J Am Soc Nephrol 2019;30:170-81. [Crossref] [PubMed]
- Elmedany SM, Naga SS, Elsharkawy R, et al. Novel urinary biomarkers and the early detection of acute kidney injury after open cardiac surgeries. J Crit Care 2017;40:171-7. [Crossref] [PubMed]
- Wang Y, Bellomo R. Cardiac surgery-associated acute kidney injury: risk factors, pathophysiology and treatment. Nat Rev Nephrol 2017;13:697-711. [Crossref] [PubMed]
- Gameiro J, Fonseca JA, Neves M, et al. Acute kidney injury in major abdominal surgery: incidence, risk factors, pathogenesis and outcomes. Ann Intensive Care 2018;8:22. [Crossref] [PubMed]
- Lin J, Fernandez H, Shashaty MGS, et al. Rate of AKI Using Consensus Creatinine-Based Criteria. Clin J Am Soc Nephrol 2015;10:1723-31. [Crossref] [PubMed]
- Chawla LS, Dommu A, Berger A, et al. Urinary sediment cast scoring index for acute kidney injury: a pilot study. Nephron Clin Pract 2008;110:c145-50. [Crossref] [PubMed]
- Kashani K, Cheungpasitporn W, Ronco C. Biomarkers of acute kidney injury: the pathway from discovery to clinical adoption. Clin Chem Lab Med 2017;55:1074-89. [Crossref] [PubMed]
- Jiang L, Zhu Y, Luo X, et al. Epidemiology of acute kidney injury in intensive care units in Beijing: the multi-center BAKIT study. BMC Nephrol 2019;20:468. [Crossref] [PubMed]