Veno-venous extracorporeal membrane oxygenation rescue for pulmonary hypertension and hypoxemic respiratory failure to obesity hypoventilation syndrome: a case report
Introduction
The effect of sleep-related breathing disorders on pulmonary hypertension (PH) is usually low. However, obesity hypoventilation syndrome (OHS) contributes to PH development and is associated with an advanced severity (1). OHS is characterized by a combination of obesity [body mass index (BMI) >30 kg/m2], daytime hypercapnia [partial pressure of carbon dioxide (PaCO2) >45 mmHg], and sleep-disordered breathing without other alternative causes of hypoventilation (2). The prevalence of PH in patients with OHS ranges between 50% and 88% (3-6). Most patients with OHS experience obstructive sleep apnea (OSA) (7,8). Advanced decompensated right ventricular dysfunction with severe PH often remains a fatal condition that cannot be treated with conventional therapies. Veno-venous extracorporeal membrane oxygenation (VV-ECMO) has emerged as a therapeutic modality for the recovery of various refractory acute respiratory failure situations (9). However, few studies have reported the outcomes in patients with OHS and refractory respiratory failure treated with VV-ECMO (10). We sought to report the case of a patient with OHS, complicated with severe PH and acute respiratory failure, who underwent bridging treatment using VV-ECMO. We present the following case in accordance with the CARE reporting checklist (available at https://apm.amegroups.com/article/view/10.21037/apm-22-178/rc).
Case presentation
A 58-year-old man with a BMI of 42 kg/m2 was admitted to the cardiac intensive care unit for progressive shortness of breath. He had chronic hepatitis B without liver cirrhosis. He had never smoked. There was no family history of cardiac disease. At the time of admission, his blood pressure was 151/98 mmHg, pulse rate was 98 beats per minute, respiration rate was 22 breaths per minute, and body temperature was 37.1 ℃ but had an oxygen saturation (SpO2) of 72% on room air. Arterial blood gas analysis (ABGA) showed a pH of 7.38, PaCO2 of 72 mmHg, PaO2 of 44 mmHg, serum bicarbonate of 42 mmol/L, and SaO2 of 75%. The N-terminal pro b-type natriuretic peptide (NT-proBNP) level was 1,150 pg/mL, and the serum troponin-I level was <0.16 ng/mL. Electrocardiography showed a normal sinus rhythm without significant changes in T-wave or ST-segment. Two-dimensional transthoracic echocardiography (TTE) revealed preserved left ventricular systolic function [left ventricular ejection fraction (LVEF), 70%], and impaired relaxation with ratio between peak early mitral inflow velocity and late mitral diastolic velocity (E/A) of 0.74. The right ventricle (RV) was dilated, the systolic function was significantly reduced, and the interventricular septum was flattened, resulting in D-shaped of left ventricle. The estimated pulmonary arterial systolic pressure (PASP) was 67 mmHg (Figure 1). Chest computed tomography scan showed tiny sub-segmental atelectasis without pulmonary embolism. We diagnosed a patient with OHS, leading to PH with right heart failure and acute respiratory failure.
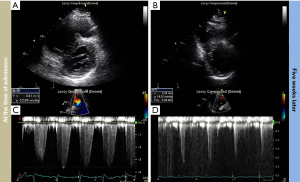
To overcome hypoxemic respiratory failure without an appropriate non-invasive ventilation (NIV) mask of helmet, a high-setting airflow of 60 L/min and a fraction of inspired oxygen (FiO2) of 80% were applied using a high-flow nasal cannula (HFNC; AIRVO2, Fisher & Paykel Healthcare, Auckland, New Zealand). The ABGA showed a pH of 7.35, PaCO2 of 84 mmHg, PaO2 of 67 mmHg, and SaO2 of 91% in the HFNC situation. He complained of rapidly worsening dyspnea and exhibited respiratory distress. He was intubated, and mechanical ventilation was applied with a tidal volume of 420 mL (6 mL/kg of predicted body weight) and positive end expiratory pressure (PEEP) of 12 cmH2O. On the first hospital day, PaCO2 of 60 mmHg and PaO2 of 48 mmHg were observed for a FiO2 of 100%. Mean arterial pressure was decreased to 50 mmHg and patient required infusion of norepinephrine at 0.3 mcg/kg/min. Extracorporeal life support with VV-ECMO was performed. An ECMO system (Capiox Emergency Bypass System, EBS; Terumo Inc., Tokyo, Japan) was performed with a 21-French drainage cannula through left femoral vein and a same sized return cannula was inserted through right femoral vein into right atrium. The initial settings of ECMO was started at a flow 4 L/kg/min, speed of 2,600 rotations/min, FiO2 of 100%, and sweep gas flow of 4 L/min. No intravenous anticoagulants were administered because of transient gastrointestinal bleeding through the nasogastric tube. Meticulous diuretics and nutritional controls were provided for weight loss during the VV-ECMO period. During ECMO period, a net fluid balance of −10,050 mL was achieved and less than 1,500 calories per day were supplied by parenteral and enteral nutrition. ECMO was successfully weaned on day 19. On day 26, the endotracheal tube was removed, and nocturnal NIV was started. After 4 weeks of hospitalization, the BMI steadily decreased from 42 to 32 kg/m2. The patient was then transferred to the rehabilitation ward.
At resting condition, pulmonary function tests showed a reduced diffusing capacity of the lungs for carbon monoxide (DLCO) of 59% with a mild restrictive pattern. The forced expiratory volume in one second (FEV1) was 2.7 L (76%), forced vital capacity (FVC) was 3.5 (74%), and FEV1/FVC was 79%. Polysomnography revealed severe OSA (Figure 2). After 5 weeks, TTE showed decreased right ventricular size with improved right ventricular systolic function and a markedly reduced PASP of 24 mmHg. The patient was discharged while maintaining nocturnal NIV, and the final daytime ABGA showed a pH of 7.38, PaCO2 of 49 mmHg, PaO2 of 60 mmHg, and SaO2 of 89% on room air.
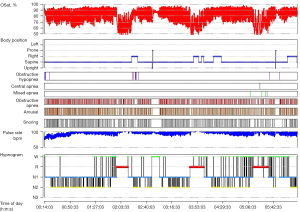
All procedures performed in this study were in accordance with the ethical standards of the institutional and/or national research committee(s) and with the Helsinki Declaration (as revised in 2013). Written informed consent was obtained from the patient for publication of this case report and accompanying images. A copy of the written consent is available for review by the editorial office of this journal.
Discussion
Unlike other sleep-related breathing disorders, OHS is closely associated with pulmonary vascular diseases, including PH (1-5). Consequences of sleep-related hypoventilation on pulmonary hemodynamics remain uncertain. Recent data suggest that sleep-related hypoventilation results mainly from nocturnal hypoxemia, and alveolar hypoventilation during sleep is responsible for the severity of PH (1). PH developed by OHS is clinically classified as PH due to lung disease and/or hypoxia (PH-lung) (11). Although the PH-lung had a severely low 3-year survival rate of 41% for chronic obstructive pulmonary disease and 16% for interstitial lung disease, the 3-year survival rate was 90% for sleep disorders/alveolar hypoventilation (12).
Although right heart catheterization (RHC) is the gold standard for determining the presence of PH, it can be used as an alternative assessment of PH (13,14). PH was defined as mean pulmonary arterial pressure (PAP) ≥25 mmHg, measured by RHC, and the recently revised cut-off value of mean PAP was >20 mmHg (11). A PASP >40 mmHg estimated by TTE was consistent with a mean PAP of ≥25 mmHg (6,13). In this case, the NIV-naïve subject with OHS exhibited crucial right ventricular failure with elevated PASP up to 67 mmHg.
The use of VV-ECMO as a bridging therapy for recovery and support for patients with refractory respiratory failure is steadily increasing. Deoxygenated blood is expelled from the body using an external centrifugal pump and then returned to the patient’s pulmonary vasculature through an appropriately oxygenated and ventilated membrane oxygenator (9). There are no medical options available to manage OHS patients with severe respiratory failure effectively. Weight reduction and volume control improve the physiological aspects of OHS, including vital capacity, voluntary ventilation, diffusion capacity, and sleep-related hypoventilation (15,16). After 4 weeks of hospitalization, intensive diuretic management and strict nutritional control were used to reduce the weight from 129 to 98 kg. VV-ECMO and mechanical ventilation were weaned after a bodyweight reduction of approximately 30 kg and a BMI decrease of 10 kg/m2 within the intensive care unit.
NIV and continuous positive airway pressure (CPAP) in patients with OHS and concomitant OSA showed improved clinical symptoms and cardiac functions, including PH (7,17). In our patient, polysomnography showed serious nocturnal hypoventilation and hypoxemia. We applied nocturnal NIV immediately after weaning from mechanical ventilation.
A limitation of this study is that RHC could not be recorded before patient deterioration. The patient progressed rapidly then underwent mechanical ventilation and VV-ECMO; thus, RHC could not be performed. RHC was not performed because cardiac function and PASP could be evaluated by TTE before intubation, and right ventricular function and PASP showed nearly complete recovery after stabilization.
This case report documented that a patient with OHS, with acute right ventricular failure and reversible pulmonary hypertensive crisis, could recover with rescue treatment using VV-ECMO for hypoxemic and hypercapnic respiratory failure.
Acknowledgments
We would like to thank Editage (https://www.editage.co.kr) for English language editing. And this study was granted only by INHA University College of Medicine.
Funding: None.
Footnote
Reporting Checklist: The authors have completed the CARE reporting checklist. Available at https://apm.amegroups.com/article/view/10.21037/apm-22-178/rc
Peer Review File: Available at https://apm.amegroups.com/article/view/10.21037/apm-22-178/prf
Conflicts of Interest: All authors have completed the ICMJE uniform disclosure form (available at https://apm.amegroups.com/article/view/10.21037/apm-22-178/coif). The authors have no conflicts of interest to declare.
Ethical Statement: The authors are accountable for all aspects of the work in ensuring that questions related to the accuracy or integrity of any part of the work are appropriately investigated and resolved. All procedures performed in this study were in accordance with the ethical standards of the institutional and/or national research committee(s) and with the Helsinki Declaration (as revised in 2013). Written informed consent was obtained from the patient for publication of this case report and accompanying images. A copy of the written consent is available for review by the editorial office of this journal.
Open Access Statement: This is an Open Access article distributed in accordance with the Creative Commons Attribution-NonCommercial-NoDerivs 4.0 International License (CC BY-NC-ND 4.0), which permits the non-commercial replication and distribution of the article with the strict proviso that no changes or edits are made and the original work is properly cited (including links to both the formal publication through the relevant DOI and the license). See: https://creativecommons.org/licenses/by-nc-nd/4.0/.
References
- Adir Y, Humbert M, Chaouat A. Sleep-related breathing disorders and pulmonary hypertension. Eur Respir J 2021;57:2002258. [Crossref] [PubMed]
- Sivam S, Yee B, Wong K, et al. Obesity Hypoventilation Syndrome: Early Detection of Nocturnal-Only Hypercapnia in an Obese Population. J Clin Sleep Med 2018;14:1477-84. [Crossref] [PubMed]
- Sugerman HJ, Baron PL, Fairman RP, et al. Hemodynamic dysfunction in obesity hypoventilation syndrome and the effects of treatment with surgically induced weight loss. Ann Surg 1988;207:604-13. [Crossref] [PubMed]
- Mokhlesi B, Tulaimat A. Recent advances in obesity hypoventilation syndrome. Chest 2007;132:1322-36. [Crossref] [PubMed]
- Alawami M, Mustafa A, Whyte K, et al. Echocardiographic and electrocardiographic findings in patients with obesity hypoventilation syndrome. Intern Med J 2015;45:68-73. [Crossref] [PubMed]
- Almeneessier AS, Nashwan SZ, Al-Shamiri MQ, et al. The prevalence of pulmonary hypertension in patients with obesity hypoventilation syndrome: a prospective observational study. J Thorac Dis 2017;9:779-88. [Crossref] [PubMed]
- Masa JF, Corral J, Alonso ML, et al. Efficacy of Different Treatment Alternatives for Obesity Hypoventilation Syndrome. Pickwick Study. Am J Respir Crit Care Med 2015;192:86-95. [Crossref] [PubMed]
- Teichtahl H. The obesity-hypoventilation syndrome revisited. Chest 2001;120:336-9. [Crossref] [PubMed]
- Abrams D, Brodie D. Extracorporeal Membrane Oxygenation for Adult Respiratory Failure: 2017 Update. Chest 2017;152:639-49. [Crossref] [PubMed]
- Umei N, Ichiba S. Venovenous Extracorporeal Membrane Oxygenation as a Treatment for Obesity Hypoventilation Syndrome. Case Rep Crit Care 2017;2017:9437452. [Crossref] [PubMed]
- Simonneau G, Montani D, Celermajer DS, et al. Haemodynamic definitions and updated clinical classification of pulmonary hypertension. Eur Respir J 2019;53:1801913. [Crossref] [PubMed]
- Hurdman J, Condliffe R, Elliot CA, et al. ASPIRE registry: assessing the Spectrum of Pulmonary hypertension Identified at a REferral centre. Eur Respir J 2012;39:945-55. [Crossref] [PubMed]
- Fisher MR, Forfia PR, Chamera E, et al. Accuracy of Doppler echocardiography in the hemodynamic assessment of pulmonary hypertension. Am J Respir Crit Care Med 2009;179:615-21. [Crossref] [PubMed]
- Howard LS, Grapsa J, Dawson D, et al. Echocardiographic assessment of pulmonary hypertension: standard operating procedure. Eur Respir Rev 2012;21:239-48. [Crossref] [PubMed]
- Olson AL, Zwillich C. The obesity hypoventilation syndrome. Am J Med 2005;118:948-56. [Crossref] [PubMed]
- Powers MA. The obesity hypoventilation syndrome. Respir Care 2008;53:1723-30. [PubMed]
- Masa JF, Mokhlesi B, Benítez I, et al. Echocardiographic Changes with Positive Airway Pressure Therapy in Obesity Hypoventilation Syndrome. Long-Term Pickwick Randomized Controlled Clinical Trial. Am J Respir Crit Care Med 2020;201:586-97. [Crossref] [PubMed]