A narrative review of positive regulation of NLRP3 inflammasome in rheumatoid arthritis
Introduction
Rheumatoid arthritis (RA) is a severe, chronic autoimmune disease that causes joint dysfunction and affects the quality of life of about 1% of the global population (1,2). RA is characterized by inflammatory synovitis, cartilage damage, bone tissue destruction, and periarticular decalcification (2,3), resulting in complications such as cardiovascular disease (4). Genetics and the environment are known to be important risk factors for triggering the disease (5). However, its pathogenesis is not completely clear. is not completely clear. Extensive inflammatory synovitis is a major pathological manifestation of RA (6), and if not treated adequately, will bring irreversible lifelong disability to patients. Disappointingly, the current diagnosis and treatment of RA is not ideal, and biotherapy also increases the risk of patient infection (7). Therefore, the development of new therapeutic strategies is particularly critical.
Inflammasome is a polyprotein complex composed of sensor, pattern recognition receptors (PRRs) and pro-caspase-1 in the cytoplasm, with a molecular weight of about 700 KDa (8). PRRs are an important part of the natural immune system, and the assembly of inflammasome is the recognition response of PRRs to pathogen-associated molecular pattern (PAMP) or damage-associated molecular pattern (DAMP) (9). Stimulated by various exogenous and endogenous pathogens or danger signals, the inflammasome induces self-shearing and activation of caspase-1, which then cleaves pro-cytokine interleukin (IL)-1β and pro-IL-18 to form mature IL-1β and IL-18 (10,11), triggering the host’s inflammatory response, and helping maintain the mechanism of tissue homeostasis and repair. RA is one of the most common inflammatory diseases, with increased concentrations of many inflammatory cytokines in its serum, most notably tumor necrosis factor (TNF), IL-6, and IL-1β (12). Extensive expression of IL-1β in the synovium allows for the activation of chondrocytes and osteoclasts, resulting in cartilage erosion and bone shedding, which is correlated with higher IL-18 levels in the synovium (13).
While inflammasome can recognize host pathogens, its dysregulation may cause excessive inflammation and unnecessary tissue damage to the body. For example, an increase in inflammasome is considered a marker of cellular senescence (14). Excessive inflammasome activation contributes to the development of malignant tumors, diabetic metabolic disorders, neurodegenerative diseases, and autoimmune diseases (15,16). In RA chronic autoimmune diseases, inflammasome triggers innate immune defense of the host by releasing pro-inflammatory cytokines such as IL-1β, and inhibition of IL-1 reduces RA symptoms and tissue radiation damage (17). NOD-like receptor (NLR) family proteins belong to PRRs (18), and at least 22 subtypes to members (19) have been identified. The NOD-like receptor family pyrin domain containing 3 (NLRP3) inflammasome is the most widely studied (20), and triggers host autoimmune defenses by recognizing foreign danger signals. In addition, studies of gene transcription or protein expression in the NLRP3 inflammasome signaling pathway (21) strongly suggest that NLRP3 inflammasome may be a clinical therapeutic target for inflammatory diseases such as RA. This review will provide a focus on the available knowledge about the NLRP3 inflammasome role in RA so that new therapies can be created for RA patients. We present the following article in accordance with the Narrative Review reporting checklist (available at https://dx.doi.org/10.21037/apm-21-3472).
NLRP3 inflammasome
NLRP3 inflammasome mainly exists in macrophages, neutrophils, and other immune cells, and is a complex multi-protein complex (22). Its structure is composed of an NLRP3 receptor, apoptosis-associated speck-like protein (ASC), and pro-caspase-1 (23). The NLRP3 receptor consists of an N-terminal pyrin domain (PYD), nucleotide triphosphatase (NACHT) domain, and C-terminal leucine-rich repeat (LRR) (24). The PYD domain mediates the interaction with ASC, leading to inflammasome assembly, and the NOD domain has ATPase activity, which can promote the autooligomerization of NLRP3. The LRR domain is an essential part of NLRP3 inflammasome activation and interacts with ligands to regulate NLRP3 activity, but its specific function remains to be clarified (25-28). NLRP3 can be activated in vivo by a variety of stimuli, such as reactive oxygen species (ROS), adenosine triphosphate (ATP), PM 2.5, bacterial, and fungal components (29-31).
Activation of NLRP3 inflammasome
Activation of NLRP3 inflammasome usually depends on extracellular stimulation, which leads to transcriptional induction of NLRP3 and post-translational modification of receptor activation. In general, classic NLRP3 inflammasome activation requires two independent and parallel signals (32). The first is the NLRP3 inflammasome initiation signal, which is triggered by endogenous molecules such as TNF or microbial components such as lipopolysaccharides (LPS). These compounds activate their homologous cytokine receptors, resulting in increased expression of NLRP3, pro-IL-1β, and pro-IL-18 dependent on NF-κB (33). The second signal is direct activation of NLRP3 inflammasome and may involve a variety of upstream cellular signaling events, such as potassium outflow, calcium signaling changes, mitochondrial dysfunction, increased ROS, and release of trypsin from lysosomal leakage (34). In addition, purinergic receptor P2X-purinoceptor-7 (P2X7), an extracellular ATP-regulated ligand-gated ion channel, also activates NLRP3 inflammasome. In the classical NLRP3 signaling pathway, activation of NLRP3 leads to the assembly of a multiprotein complex that recruits caspase-1 by interacting with the ASC and NACHT domains to promote caspase-1 activation. Subsequently, activated caspase-1 cleaves pro-IL-1β and pro-IL-18 into their active forms (35,36), thereby inducing an inflammatory response (Figure 1). Assembly of inflammasome results from activation of enzyme function, primarily casparase-1. An important manifestation of NLRP3 inflammasome activation is the release of pro-IL-1β and pro-IL-18, which are critical for inflammatory response and immune function.
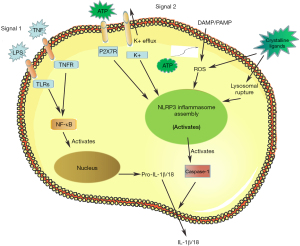
NLRP3 inflammasome can also be activated through a non-classical signaling pathway considered as a preferred response to gram-negative bacteria (37), which may not play a role in gram-positive bacterial infections. The non-classical pathway involves activation of mouse caspase-11, which is triggered by intracellular LPS, which indirectly promotes IL-1β secretion by triggering the standard NLRP3 inflammasome (38), although the association between caspase-11 and NLRP3 activation remains unclear. Direct binding of LPS to mouse caspase-11 and human caspase-4 and caspase-5 induces activation of atypical signaling pathways (39).
NLRP3 inflammasome and RA
RA is a chronic autoinflammatory arthritis that may be associated with genetic and environmental triggers, leading to joint malformations and dysfunction. In irreversible joint destruction and synovitis, even can produce motor injury and premature death. The NLRP3 inflammasome is primarily involved in the pathogenesis and development of RA, and the polymorphisms of NLRP3 gene indirectly respond to the incidence and pathological severity of RA.
Clinical studies have found that NLRP3 expression is upregulated at the mRNA and protein levels in monocytes, macrophages, centriophils, and dendritic cells in RA patients (12,40,41), suggesting that NLRP3 activation is involved in systemic or local inflammation of RA. In other cell types, the investigators obtained the opposite results. In neutrophils, NLRP3 and ASC expression were significantly downregulated at the mRNA and protein levels. Furthermore, the serum IL-18 levels increased with the activated caspase-1, while IL-1 did not. Meanwhile, NLRP3 mRNA levels were inversely associated with disease severity in the neutrophils of RA patients. These results suggest that hyperactivation of caspase-1 in neutrophils in RA patients may mediate IL-18 activation to promoting disease progression in an NLRP3 inflammasome-independent manner, suggesting a protective role of NLRP3 inflammasome in RA. In particular, myeloid and endothelial cells showed enhanced NLRP3, ASC, and caspase-1 expression and were considered to be major sources of IL-1β secretion in synovium (42). However, the present evidence does not fully explain the pathophysiology of the NLRP3 inflammasome in RA, or whether it is activated during synovial inflammation.
In vivo studies tell us that the expression of NLRP3 inflammasome components is enhanced in macrophages, and the lack of NLRP3 suppresses the development of arthritis and cartilage destruction (43). The expression of NLRP3, caspase-1, and IL-1 was increased in the serum and knee synovium of collagen (CIA) mice, and the animals lacking IL-18 gene expression were less vulnerable to CIA-induced arthritis (44). Higher levels of NLRP3, ASC, caspase-1, and pro-IL-1 were observed in the basal cells of RA patients (45,46). A study (47) of myeloid cell-specific A20−/− mice showed that an improvement in arthritis was observed in mice after loss of NLRP3, caspasase-1/11, or IL-1 receptor (IL-1R). ASC knockout inhibited virtually all typical inflammatory bodies, protecting mice from arthritis in collagen-induced arthritis models (48) and alleviating arthritis symptoms in antigen-induced arthritis models (49).
NLRP3 inflammasome as a promising target in RA therapy
Abnormal activation of NLRP3 inflammasome plays a crucial role in the pathogenesis of inflammation and autoimmune, and inhibition of NLRP3 inflammasome is expected to be an effective strategy for the treatment of RA, which has aroused great interest. Considering the intrinsic mechanism of NLRP3 in RA, several NLRP3 inflammasome inhibitors have been identified, including one that directly inhibits NLRP3 protein and another that indirectly inhibits caspase-1 and IL-1(IL-1β)/IL-18 signaling pathways (Figure 2), which may pave the way for new treatments for RA.
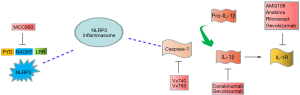
NLRP3 inhibitors
The small molecule compound MCC950 is currently the most potent and highly specific inhibitor of NLRP3 inflammasome (50). MCC950 blocks ATP hydrolysis and inhibits NLRP3 inflammasome formation and activation by directly interacting with the NACHT domain (51,52). Before MCC950 was identified as an NLRP3 targeting molecule, it was found to be an inhibitor of IL-1β activation (50). MCC950 was also shown to reduce the severity of CIA, lupus nephritis (LN), and experimental autoimmune encephalomyelitis (EAE) mice (44,50,53), which led to the beginning of a series of clinical trials. Unfortunately, testing for phase II-RA was suspended due to drug toxicity (36). Similar to MCC950, tranilast was found to bind to the NACHT domain of NLRP3. In a mouse RA model, treatment with tranilast reduced CIA-induced arthritis (54). Although its mode of action is not known, the adverse effects of tranilast are very low, so in-depth study of its effectiveness and specificity in inhibiting NLRP3 may provide a new therapeutic tool for the treatment of complex diseases in which NLRP3 is involved. Moreover, compound CY-09 specifically binds to the ATPase domain of NLRP3, thereby blocking the oligomerization of NLRP3 (55). CY-09 was effective in reducing inflammation in CAPS mouse models. It has also been found in vitro to reduce IL-1β secretion in synovial fluid cells (SFCs) of gout patients (55). Therefore, CY-09 may be a candidate for further study of RA. miRNAs bind to a conserved site in the 3 'UTR of NLRP3 transcripts and provide an additional pathway to inhibit NLRP3 inflammasome. miRNA-20a exerts anti-inflammatory effects by inhibiting NLRP3 protein expression in RA and systemic lupus erythematosus (SLE) mouse models (56). We also found that some other drugs (such as isoflurane, dopamine (DA), P2X7, Bay11-7082 and parthenolactone, etc.) inhibit NLRP3 inflammasome activation (57-60), but their clinical application in RA needs further study.
IL-1(IL-1β) or IL-18 inhibitors
IL-1β and IL-18 are the major products of the activated NLRP3 inflammasome, and are involved in the pathogenesis of inflammatory or autoimmune-related diseases. Therefore, IL-1β/IL-18 blockade is a prominent therapeutic strategy that has been used in several NLRP3-mediated diseases. Currently, three drugs have been approved for clinical use: Anakinra; Canakinumab; Rilonacept. Anakinra, a recombinant IL-1R antagonist, has been clinically used in RA and autoinflammatory diseases for nearly 20 years (61,62). However, with a short plasma half-life (4–6 hours), Anakinra must be injected daily. Therefore, a half-life (26 days) with longer Canakinumaban (IL-1β-neutralizing antibody) is a better treatment option (63). Recombinant protein Rilonacept is an alternative to Anakinra, a decoy receptor for IL-1α and IL-1β, which is clinically effective for gout (64).
Furthermore, other biologics were developed to block the IL-1 pathway, but have not been used in clinical practice. The application of IL-18 blocking antibody GSK1070806 is only in the early stage (23); IL-1β antagonist antibody Gevokizumab strongly binds to IL-1β without inhibiting its recruitment to IL-1R (65); AMG108 antibody binds and neutralizes IL-1R, leading to the complete failure of IL-1α and IL-1β signaling pathways (66).
Caspase-1 inhibitors
As the protease caspase-1 performs the enzymatic activity of the inflammasome, which makes intervention in caspase-1 an attractive target for pharmacological intervention, but no drug based on caspase inhibition has been approved (67). In the mouse model, inhibition of caspase-1 was significantly effective in RA and osteoarthritis (OA) (67). VX-740/VX-765 blocks the cleavage of pro-IL-1β/18 by catalyzing the covalent modification of the cysteine residues at the caspase-1 active site (68). Among these, VX-740 reduces joint damage in both RA and OA mice (69). VX-740 has entered a phase II clinical trial of RA and is withdrawn due to hepatotoxicity (67,70). Furthermore, it was found for VX-765 to reduce IL-1β and IL-18 release in mouse models, thereby improving the severity of RA and psoriasis (71).
Conclusions
Increasingly, studies have shown that inflammasome plays an important role in inflammation and other diseases and is a promising target for pathological treatment. Therefore, it is important to understand the dynamic balance between the beneficial and harmful activation of inflammasome, especially with a view to understanding its mechanisms. As a member of the inflammasome family, activation of NLRP3 inflammasome is responsible for a variety of autoimmune diseases, including RA, and its regulation of downstream molecules (caspase-1, IL-1β, and IL-18) has been well documented. In many diseases, targeted treatment can be achieved with IL-1β or IL-18 inhibitors, and ethnic differences in NLRP3-related gene polymorphisms have been found, suggesting that targeting NLRP3 may be more advantageous in the future than using IL-1β or inhibitors (72). Although researchers have improved their understanding of the molecular activation mechanisms of NLRP3 inflammasome over the past few decades, many aspects remain to be addressed. In conclusion, the occurrence of NLRP3 inflammasome in RA and other autoimmune diseases is a promising therapeutic target for the development of effective therapeutic strategies for related inflammatory diseases in the future.
Acknowledgments
Funding: None.
Footnote
Reporting Checklist: The authors have completed the Narrative Review reporting checklist. Available at https://dx.doi.org/10.21037/apm-21-3472
Conflicts of Interest: All authors have completed the ICMJE uniform disclosure form (available at https://dx.doi.org/10.21037/apm-21-3472). The authors have no conflicts of interest to declare.
Ethical Statement: The authors are accountable for all aspects of the work in ensuring that questions related to the accuracy or integrity of any part of the work are appropriately investigated and resolved.
Open Access Statement: This is an Open Access article distributed in accordance with the Creative Commons Attribution-NonCommercial-NoDerivs 4.0 International License (CC BY-NC-ND 4.0), which permits the non-commercial replication and distribution of the article with the strict proviso that no changes or edits are made and the original work is properly cited (including links to both the formal publication through the relevant DOI and the license). See: https://creativecommons.org/licenses/by-nc-nd/4.0/.
References
- Sardar S, Kerr A, Vaartjes D, et al. The oncoprotein TBX3 is controlling severity in experimental arthritis. Arthritis Res Ther 2019;21:16. [Crossref] [PubMed]
- Rudan I, Sidhu S, Papana A, et al. Prevalence of rheumatoid arthritis in low- and middle-income countries: A systematic review and analysis. J Glob Health 2015;5:010409. [PubMed]
- McInnes IB, Schett G. The pathogenesis of rheumatoid arthritis. N Engl J Med 2011;365:2205-19. [Crossref] [PubMed]
- Firestein GS, McInnes IB. Immunopathogenesis of Rheumatoid Arthritis. Immunity 2017;46:183-96. [Crossref] [PubMed]
- Malmström V, Catrina AI, Klareskog L. The immunopathogenesis of seropositive rheumatoid arthritis: from triggering to targeting. Nat Rev Immunol 2017;17:60-75. [Crossref] [PubMed]
- Alsaleh G, François A, Knapp AM, et al. Synovial fibroblasts promote immunoglobulin class switching by a mechanism involving BAFF. Eur J Immunol 2011;41:2113-22. [Crossref] [PubMed]
- Siebert S, Tsoukas A, Robertson J, et al. Cytokines as therapeutic targets in rheumatoid arthritis and other inflammatory diseases. Pharmacol Rev 2015;67:280-309. [Crossref] [PubMed]
- Strowig T, Henao-Mejia J, Elinav E, et al. Inflammasomes in health and disease. Nature 2012;481:278-86. [Crossref] [PubMed]
- Takeuchi O, Akira S. Pattern recognition receptors and inflammation. Cell 2010;140:805-20. [Crossref] [PubMed]
- Malik A, Kanneganti TD. Inflammasome activation and assembly at a glance. J Cell Sci 2017;130:3955-63. [Crossref] [PubMed]
- de Torre-Minguela C, Mesa Del Castillo P, Pelegrín P. The NLRP3 and Pyrin Inflammasomes: Implications in the Pathophysiology of Autoinflammatory Diseases. Front Immunol 2017;8:43. [Crossref] [PubMed]
- McInnes IB, Schett G. Cytokines in the pathogenesis of rheumatoid arthritis. Nat Rev Immunol 2007;7:429-42. [Crossref] [PubMed]
- Wei XQ, Leung BP, Arthur HM, et al. Reduced incidence and severity of collagen-induced arthritis in mice lacking IL-18. J Immunol 2001;166:517-21. [Crossref] [PubMed]
- Latz E, Duewell P. NLRP3 inflammasome activation in inflammaging. Semin Immunol 2018;40:61-73. [Crossref] [PubMed]
- He Y, Hara H, Núñez G. Mechanism and Regulation of NLRP3 Inflammasome Activation. Trends Biochem Sci 2016;41:1012-21. [Crossref] [PubMed]
- Spel L, Martinon F. Inflammasomes contributing to inflammation in arthritis. Immunol Rev 2020;294:48-62. [Crossref] [PubMed]
- Bresnihan B, Alvaro-Gracia JM, Cobby M, et al. Treatment of rheumatoid arthritis with recombinant human interleukin-1 receptor antagonist. Arthritis Rheum 1998;41:2196-204. [Crossref] [PubMed]
- Haneklaus M, O'Neill LA, Coll RC. Modulatory mechanisms controlling the NLRP3 inflammasome in inflammation: recent developments. Curr Opin Immunol 2013;25:40-5. [Crossref] [PubMed]
- Mariathasan S, Weiss DS, Newton K, et al. Cryopyrin activates the inflammasome in response to toxins and ATP. Nature 2006;440:228-32. [Crossref] [PubMed]
- Shao BZ, Xu ZQ, Han BZ, et al. NLRP3 inflammasome and its inhibitors: a review. Front Pharmacol 2015;6:262. [Crossref] [PubMed]
- Latz E, Xiao TS, Stutz A. Activation and regulation of the inflammasomes. Nat Rev Immunol 2013;13:397-411. [Crossref] [PubMed]
- Kummer JA, Broekhuizen R, Everett H, et al. Inflammasome components NALP 1 and 3 show distinct but separate expression profiles in human tissues suggesting a site-specific role in the inflammatory response. J Histochem Cytochem 2007;55:443-52. [Crossref] [PubMed]
- Ozaki E, Campbell M, Doyle SL. Targeting the NLRP3 inflammasome in chronic inflammatory diseases: current perspectives. J Inflamm Res 2015;8:15-27. [PubMed]
- Saleh M. The machinery of Nod-like receptors: refining the paths to immunity and cell death. Immunol Rev 2011;243:235-46. [Crossref] [PubMed]
- Vajjhala PR, Mirams RE, Hill JM. Multiple binding sites on the pyrin domain of ASC protein allow self-association and interaction with NLRP3 protein. J Biol Chem 2012;287:41732-43. [Crossref] [PubMed]
- Duncan JA, Bergstralh DT, Wang Y, et al. Cryopyrin/NALP3 binds ATP/dATP, is an ATPase, and requires ATP binding to mediate inflammatory signaling. Proc Natl Acad Sci U S A 2007;104:8041-6. [Crossref] [PubMed]
- Ye Z, Ting JP. NLR, the nucleotide-binding domain leucine-rich repeat containing gene family. Curr Opin Immunol 2008;20:3-9. [Crossref] [PubMed]
- Hafner-Bratkovič I, Sušjan P, Lainšček D, et al. NLRP3 lacking the leucine-rich repeat domain can be fully activated via the canonical inflammasome pathway. Nat Commun 2018;9:5182. [Crossref] [PubMed]
- Cruz CM, Rinna A, Forman HJ, et al. ATP activates a reactive oxygen species-dependent oxidative stress response and secretion of proinflammatory cytokines in macrophages. J Biol Chem 2007;282:2871-9. [Crossref] [PubMed]
- Erdei J, Tóth A, Balogh E, et al. Induction of NLRP3 Inflammasome Activation by Heme in Human Endothelial Cells. Oxid Med Cell Longev 2018;2018:4310816. [Crossref] [PubMed]
- Lee MS, Kwon H, Lee EY, et al. Shiga Toxins Activate the NLRP3 Inflammasome Pathway To Promote Both Production of the Proinflammatory Cytokine Interleukin-1β and Apoptotic Cell Death. Infect Immun 2015;84:172-86. [Crossref] [PubMed]
- Lu A, Magupalli VG, Ruan J, et al. Unified polymerization mechanism for the assembly of ASC-dependent inflammasomes. Cell. 2014;156:1193-206. [Crossref] [PubMed]
- Franchi L, Eigenbrod T, Núñez G. Cutting edge: TNF-alpha mediates sensitization to ATP and silica via the NLRP3 inflammasome in the absence of microbial stimulation. J Immunol 2009;183:792-6. [Crossref] [PubMed]
- Hu Z, Yan C, Liu P, et al. Crystal structure of NLRC4 reveals its autoinhibition mechanism. Science 2013;341:172-5. [Crossref] [PubMed]
- Lu A, Li Y, Schmidt FI, et al. Molecular basis of caspase-1 polymerization and its inhibition by a new capping mechanism. Nat Struct Mol Biol 2016;23:416-25. [Crossref] [PubMed]
- Swanson KV, Deng M, Ting JP. The NLRP3 inflammasome: molecular activation and regulation to therapeutics. Nat Rev Immunol 2019;19:477-89. [Crossref] [PubMed]
- Kayagaki N, Wong MT, Stowe IB, et al. Noncanonical inflammasome activation by intracellular LPS independent of TLR4. Science 2013;341:1246-9. [Crossref] [PubMed]
- Yang D, He Y, Muñoz-Planillo R, et al. Caspase-11 Requires the Pannexin-1 Channel and the Purinergic P2X7 Pore to Mediate Pyroptosis and Endotoxic Shock. Immunity 2015;43:923-32. [Crossref] [PubMed]
- Shi J, Zhao Y, Wang Y, et al. Inflammatory caspases are innate immune receptors for intracellular LPS. Nature 2014;514:187-92. [Crossref] [PubMed]
- Paramel GV, Sirsjö A, Fransén K. Role of genetic alterations in the NLRP3 and CARD8 genes in health and disease. Mediators Inflamm 2015;2015:846782. [Crossref] [PubMed]
- Choulaki C, Papadaki G, Repa A, et al. Enhanced activity of NLRP3 inflammasome in peripheral blood cells of patients with active rheumatoid arthritis. Arthritis Res Ther 2015;17:257. [Crossref] [PubMed]
- Kolly L, Busso N, Palmer G, et al. Expression and function of the NALP3 inflammasome in rheumatoid synovium. Immunology 2010;129:178-85. [Crossref] [PubMed]
- Vande Walle L, Van Opdenbosch N, Jacques P, et al. Negative regulation of the NLRP3 inflammasome by A20 protects against arthritis. Nature 2014;512:69-73. [Crossref] [PubMed]
- Guo C, Fu R, Wang S, et al. NLRP3 inflammasome activation contributes to the pathogenesis of rheumatoid arthritis. Clin Exp Immunol 2018;194:231-43. [Crossref] [PubMed]
- Mathews RJ, Robinson JI, Battellino M, et al. Evidence of NLRP3-inflammasome activation in rheumatoid arthritis (RA); genetic variants within the NLRP3-inflammasome complex in relation to susceptibility to RA and response to anti-TNF treatment. Ann Rheum Dis 2014;73:1202-10. [Crossref] [PubMed]
- Addobbati C, da Cruz HLA, Adelino JE, et al. Polymorphisms and expression of inflammasome genes are associated with the development and severity of rheumatoid arthritis in Brazilian patients. Inflamm Res 2018;67:255-64. [Crossref] [PubMed]
- Fusco R, Siracusa R, Genovese T, et al. Focus on the Role of NLRP3 Inflammasome in Diseases. Int J Mol Sci 2020;21:4223. [Crossref] [PubMed]
- Ippagunta SK, Brand DD, Luo J, et al. Inflammasome-independent role of apoptosis-associated speck-like protein containing a CARD (ASC) in T cell priming is critical for collagen-induced arthritis. J Biol Chem 2010;285:12454-62. [Crossref] [PubMed]
- Kolly L, Karababa M, Joosten LA, et al. Inflammatory role of ASC in antigen-induced arthritis is independent of caspase-1, NALP-3, and IPAF. J Immunol 2009;183:4003-12. [Crossref] [PubMed]
- Coll RC, Robertson AA, Chae JJ, et al. A small-molecule inhibitor of the NLRP3 inflammasome for the treatment of inflammatory diseases. Nat Med 2015;21:248-55. [Crossref] [PubMed]
- Coll RC, Hill JR, Day CJ, et al. MCC950 directly targets the NLRP3 ATP-hydrolysis motif for inflammasome inhibition. Nat Chem Biol 2019;15:556-9. [Crossref] [PubMed]
- Tapia-Abellán A, Angosto-Bazarra D, Martínez-Banaclocha H, et al. MCC950 closes the active conformation of NLRP3 to an inactive state. Nat Chem Biol 2019;15:560-4. [Crossref] [PubMed]
- Fu R, Guo C, Wang S, et al. Podocyte Activation of NLRP3 Inflammasomes Contributes to the Development of Proteinuria in Lupus Nephritis. Arthritis Rheumatol 2017;69:1636-46. [Crossref] [PubMed]
- Huang Y, Jiang H, Chen Y, et al. Tranilast directly targets NLRP3 to treat inflammasome-driven diseases. EMBO Mol Med 2018;10:e8689. [Crossref] [PubMed]
- Jiang H, He H, Chen Y, et al. Identification of a selective and direct NLRP3 inhibitor to treat inflammatory disorders. J Exp Med 2017;214:3219-38. [Crossref] [PubMed]
- Li XF, Shen WW, Sun YY, et al. MicroRNA-20a negatively regulates expression of NLRP3-inflammasome by targeting TXNIP in adjuvant-induced arthritis fibroblast-like synoviocytes. Joint Bone Spine 2016;83:695-700. [Crossref] [PubMed]
- Zahid A, Li B, Kombe AJK, et al. Pharmacological Inhibitors of the NLRP3 Inflammasome. Front Immunol 2019;10:2538. [Crossref] [PubMed]
- Yuan Y, Liu Z. Isoflurane attenuates murine lupus nephritis by inhibiting NLRP3 inflammasome activation. Int J Clin Exp Med 2015;8:17730-8. [PubMed]
- Zhao J, Wang H, Dai C, et al. P2X7 blockade attenuates murine lupus nephritis by inhibiting activation of the NLRP3/ASC/caspase 1 pathway. Arthritis Rheum 2013;65:3176-85. [Crossref] [PubMed]
- Zhao J, Zhang H, Huang Y, et al. Bay11-7082 attenuates murine lupus nephritis via inhibiting NLRP3 inflammasome and NF-κB activation. Int Immunopharmacol 2013;17:116-22. [Crossref] [PubMed]
- Calabrese LH. Anakinra treatment of patients with rheumatoid arthritis. Ann Pharmacother 2002;36:1204-9. [Crossref] [PubMed]
- Dein E, Ingolia A, Connolly C, et al. Anakinra for Recurrent Fevers in Systemic Lupus Erythematosus. Cureus 2018;10:e3782. [Crossref] [PubMed]
- Chakraborty A, Tannenbaum S, Rordorf C, et al. Pharmacokinetic and pharmacodynamic properties of canakinumab, a human anti-interleukin-1β monoclonal antibody. Clin Pharmacokinet 2012;51:e1-18. [Crossref] [PubMed]
- Schumacher HR Jr, Sundy JS, Terkeltaub R, et al. Rilonacept (interleukin-1 trap) in the prevention of acute gout flares during initiation of urate-lowering therapy: results of a phase II randomized, double-blind, placebo-controlled trial. Arthritis Rheum 2012;64:876-84. [Crossref] [PubMed]
- Geiler J, McDermott MF. Gevokizumab, an anti-IL-1β mAb for the potential treatment of type 1 and 2 diabetes, rheumatoid arthritis and cardiovascular disease. Curr Opin Mol Ther 2010;12:755-69. [PubMed]
- Cardiel MH, Tak PP, Bensen W, et al. A phase 2 randomized, double-blind study of AMG 108, a fully human monoclonal antibody to IL-1R, in patients with rheumatoid arthritis. Arthritis Res Ther 2010;12:R192. [Crossref] [PubMed]
- Kudelova J, Fleischmannova J, Adamova E, et al. Pharmacological caspase inhibitors: research towards therapeutic perspectives. J Physiol Pharmacol 2015;66:473-82. [PubMed]
- Cornelis S, Kersse K, Festjens N, et al. Inflammatory caspases: targets for novel therapies. Curr Pharm Des 2007;13:367-85. [Crossref] [PubMed]
- Rudolphi K, Gerwin N, Verzijl N, et al. Pralnacasan, an inhibitor of interleukin-1beta converting enzyme, reduces joint damage in two murine models of osteoarthritis. Osteoarthritis Cartilage 2003;11:738-46. [Crossref] [PubMed]
- Strand V, Sokolove J. Randomized controlled trial design in rheumatoid arthritis: the past decade. Arthritis Res Ther 2009;11:205. [Crossref] [PubMed]
- Zhang Y, Zheng Y. Effects and mechanisms of potent caspase-1 inhibitor VX765 treatment on collagen-induced arthritis in mice. Clin Exp Rheumatol 2016;34:111-8. [PubMed]
- Liu L, Dong Y, Ye M, et al. The Pathogenic Role of NLRP3 Inflammasome Activation in Inflammatory Bowel Diseases of Both Mice and Humans. J Crohns Colitis 2017;11:737-50. [PubMed]
(English Language Editor: B. Draper)