Expression profile of circular RNAs in oral lichen planus
Introduction
Oral lichen planus (OLP) is a common chronic inflammatory disease that affects the skin, genitalia, and oral mucous membrane. The prevalence of OLP is 1–2% (1), and it often occurs in middle-aged people, especially women (2). Most OLP patients suffer pain or other uncomfortable sensations when the lesioned areas are touched (3). The etiology and pathogenesis of OLP remain unclear, but many studies have reported that it is a T-lymphocyte-mediated autoimmune disease (4). The probability of OLP transforming into oral squamous cell carcinoma is about 1–2% (5). OLP has been classified as a potentially malignant disorder by the World Health Organization. Thus, the prevention and early treatment of OLP have considerable clinical significance.
Circular RNA (circRNA) is a popular new area of RNA research. CircRNA is produced by the back splicing of precursor messenger RNA in eukaryotes (6). CircRNAs are expressed in cell-type and tissue-type specific patterns and occur widely in eukaryotes (6-8). Unlike linear RNAs, circRNAs have no 5' cap structure or 3' adenylate tail. Further, as they cannot be broken down by ribonuclease, which is an exonuclease enzyme that degrades linear RNA molecules, they are more stable than linear RNAs (6). Most circRNAs are exonic; however, intronic circRNAs, intergenic circRNAs, antisense circRNAs, or sense overlapping circRNAs also exist (9,10).
Since Hansen et al. (11) first published a paper on the function of circRNA in the Nature journal in 2013, an increasing number of studies have shown that circRNAs play important roles in the pathogenesis of diseases and are potential molecular markers (12,13). By binding micro (mi)RNA through miRNA response elements, circRNAs act as “miRNA sponges” to undermine the inhibitory effects of miRNAs on their target mRNAs and thus regulate the expression level of mRNAs (14-16). The expression of circRNAs in the process of immune response and immune-related diseases can be altered (17). Additionally, circRNAs can promote immune responses and affect the process of autoimmune diseases, tumor immunity, and antiviral immunity (18).
Thus, circRNAs play important roles in regulating gene expression and participating in the pathogenesis of many diseases. However, the expression profile of circRNAs in OLP has not yet been determined. This study sought to identify the circRNAs differentially expressed in OLP to investigate the possible role of circRNAs in this disease’s occurrence and development.
We present the following article in accordance with the MDAR checklist (available at http://dx.doi.org/10.21037/apm-20-2253).
Methods
Patients and samples
Biopsies of oral mucosa were collected from 6 OLP patients who were clinically and pathologically diagnosed with OLP at the Department of Oral Mucosa, The Ninth People’s Hospital of Shanghai, Jiaotong University, School of Medicine (Shanghai, China) between September and October 2018. Six normal oral mucosae samples (without inflammation) were collected as controls samples from patients who underwent plastic surgery in the Plastic Surgery Department (see Table 1). All of the samples were stored at −80 °C until RNA extraction. None of the patients had used local or systemic glucocorticoids or had suffered from severe cardiovascular system disease or any liver, kidney, or other organ dysfunction. All of the patients participated voluntarily in this study and signed informed consent forms before the surgery. The study was approved by the Ethics Committee of The Ninth People’s Hospital of Shanghai [No. 89 (2012) 21]. The study was conducted in accordance with the Declaration of Helsinki (as revised in 2013).
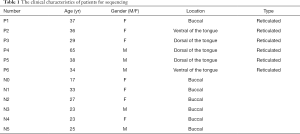
Full table
RNA isolation and quality control
Trizol (Life Technologies, Carlsbad, CA, USA) isolated total RNA from the OLP and normal oral mucosal tissues. The NanoDrop ND-1000 (Thermo Fisher Scientific, Waltham, MA, USA) was used to determine the purity and quantity of the isolated total RNA. The spectrophotometer OD260/OD280 value was used as the RNA purity index; an OD260/OD280 value between 1.8 and 2.1 was set as the range for qualification.
RNA library construction and circRNA sequencing analysis
CloudSeq Biotech (Shanghai, China) undertook the RNA library preparation and high-throughput sequencing. Ribo-Zero rRNA Removal Kits (Illumina, USA) were used to remove ribosomal (r)RNA from total RNA for each sample. The TruSeq Stranded Total RNA Library Prep Kit (Illumina, USA) was used to construct RNA libraries for the rRNA-depleted RNAs. The BioAnalyzer 2100 system (Agilent Technologies, USA) was used to conduct quality control and quantify the sequencing libraries. A gene ontology (GO) functional analysis and Kyoto Encyclopedia of Genes and Genomes (KEGG) pathway analysis were performed to predict the functions of the circRNAs.
Reverse transcription-quantitative polymerase chain reaction (RT-qPCR)
As Table 2 shows, 10 circRNAs with significant differences were selected from the sequencing results. The selection criteria were a large logFC value, a small P-value, and an average original signal expression value. RT-qPCR verification was performed on the 12 samples. RT-qPCR verification was performed on these 12 samples. The qPCR SYBR Green master mix (Cloudseq, Shanghai, China) was used to undertake the RT-qPCR of the 10 selected circRNAs. The RNase Inhibitor (Enzymatics, Green Bay, WI, USA), SuperScriptTM III Reverse Transcriptase (Thermo Fisher Scientific, Chino, CA, USA), and dNTP Mix (HyTest Ltd., Turku, Finland) were used to construct complementary deoxyribonucleic acid (cDNA) libraries. Glyceraldehyde 3-phosphate dehydrogenase (GAPDH) was used as an endogenous reference gene. The data analysis was performed using the 2-∆∆CT method.
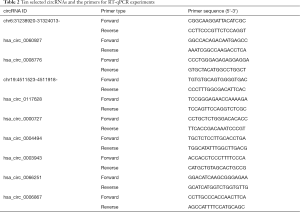
Full table
Functional enrichment analysis and ceRNA network analysis
A GO functional analysis and a KEGG pathway analysis were performed on the host genes of abnormally expressed circRNAs to predict these circRNAs’ function and identify the possible pathways involved. The GO analysis mainly examined the biological processes, cellular components, and molecular functions of the circRNAs. TargetScan and miRanda were used to predict the target miRNA of abnormally expressed circRNAs, and Cytoscape (v2.8.0) software was used to construct a ceRNA network map.
Statistical analysis
Graphpad Prism version 8.0 and statistical software SPSS 19.0 (SPSS, Chicago, IL, USA) was used for the statistical analysis. The quantitative data were expressed as mean ± standard deviation (SD). Unpaired t-tests analyzed differences between the two groups.
Expression profile and characteristics of differentially expressed circRNAs between OLP and normal oral mucosal tissues
Six oral mucosal tissues from untreated patients with OLP and 6 normal oral mucosal tissues were used for the circRNA sequencing; 21,505 circRNA transcripts were identified, of which 15,801 were upregulated, and 5,704 were downregulated in OLP; 135 circRNAs were identified (with fold changes ≥2.0 and a P<0.05) as differentially expressed circRNAs. Eighty-three circRNAs were significantly upregulated, and 52 were markedly downregulated. A volcano plot (see Figure 1A), a scatter plot (see Figure 1B), and a hierarchical clustering heatmap (see Figure 1C) showed that the expression levels of the circRNAs differed significantly between the OLP group and normal oral mucosal group.
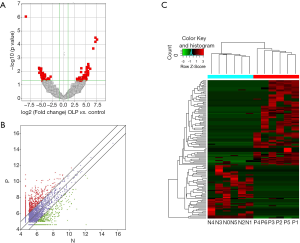
The original sequencing data have been uploaded to the GEO website (https://www.ncbi.nlm.nih.gov/geo/); the GEO serial number is GSE131567.
Among the 135 differentially expressed circRNAs, 19 were identified for the first time and deemed novel circRNAs; the remaining 116 circRNAs had been reported previously in published circRNA databases or articles (see Figure 2A). These circRNAs were located across all human chromosomes, except the Y chromosome. No circRNA located across the mitochondrial genome was found (see Figure 2B). In terms of length, 116 identified circRNAs were less than 2,000 nucleotides (nt), and 19 were more than 2,000 nt (see Figure 2C). Among the 135 identified circRNAs, 113 were exonic circRNAs, 8 were intronic circRNAs, 13 were overlapping circRNAs, and 1 was an intergenic circRNA (see Figure 2D).
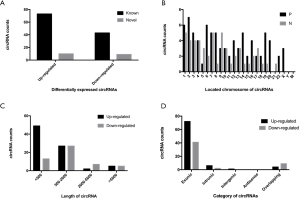
Results of RT-qPCR
Seven upregulated and three downregulated circRNAs were screened according to their fold changes and P values between the OLP and normal oral mucosal samples. These 10 significantly differentially expressed circRNAs were confirmed by RT-qPCR, and all of them showed the same significant differences as those detected in the sequencing results (see Figure 3). Chr19:4511523-4511918-, hsa_circ_0004494, chr6:31238920-31324013-, hsa_circ_0060927, hsa_circ_0008776, hsa_circ_0117628, and hsa_circ_0000727 were upregulated and hsa_circ_0006867, hsa_circ_0003943, and hsa_circ_0066251 were downregulated. Among these 10 circRNAs, chr19:4511523-4511918-, and chr6:31238920-31324013- were novel circRNAs. The biological features of these 10 circRNAs are set out in Table 3.
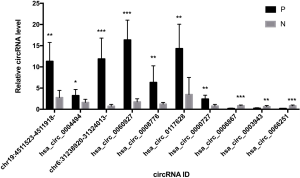
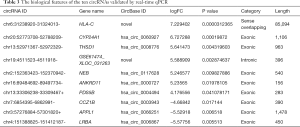
Full table
Predicted GO function and KEGG functional pathways of circRNAs in OLP
The GO enrichment analysis predicted that the target host genes’ functions were involved in biological processes, cellular components, and molecular functions. A total of 535 GO functional items were enriched. Figure 4 shows the top 10 annotation terms under which the differentially expressed circRNAs are clustered. Differentially expressed circRNAs are mainly involved in biological processes (e.g., “antigen processing and [the] presentation of endogenous peptide antigen[s],” the “positive regulation of macroautophagy,” and “antigen processing and [the] presentation of endogenous antigen[s]”), participated in the composition of cellular components (e.g., the “MHC class I protein complex”), and were involved in molecular functions (e.g., “ubiquitin-like modifier activating enzyme activity”).
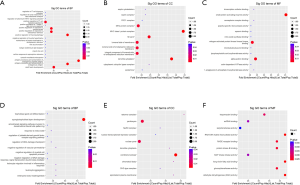
The KEGG analysis results revealed the pathways related to the functions of the target genes of the differentially expressed circRNAs (see Figure 5). A total of 78 signal pathways were identified, of which 75 were involved in upregulated genes, and 7 were involved in downregulated genes. Among these, 4 were enriched in both upregulated and downregulated genes, including the “Phospholipase D signaling pathway,” “ErbB signaling pathway,” “Ras signaling pathway,” and “Pathways in cancer.” Figure 6 shows the detailed pathway signals of the “B-cell receptor signaling pathway,” “T-cell receptor signaling pathway,” “cGMP-PKG signaling pathway,” and “phospholipase D signaling pathway.”
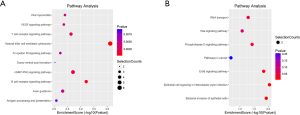
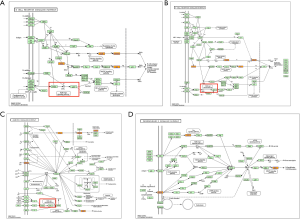
Prediction and visualization of a ceRNA network
The downstream miRNAs of the 10 circRNAs verified by RT-qPCR were predicted, and a ceRNA network map was constructed (see Figure 7). The results showed that 10 selected circRNAs all had miRNA response elements, and may bind with miRNAs; for example: chr6:31238920-31324013-, hsa_circ_0006867, hsa_circ_0066251 may bind with hsa-miR-27b-3p, Chr6:31238920-31324013- may bind with hsa-miR-802, chr6:31238920-31324013-, and hsa_circ_0060927 may bind with hsa-miR-137.
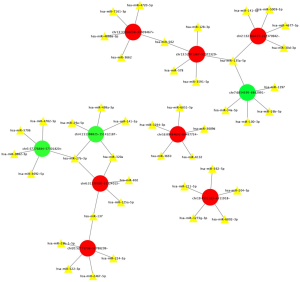
Discussion
As stated above, OLP is a common chronic inflammatory disease of the oral mucosa. In addition to the etiology and pathogenesis of OLP being complex and unknown, there is a lack of clinically effective drugs to treat OLP (2,3). Thus, the pathogenesis of OLP needs to be studied, and effective therapeutic targets for the prevention and treatment of this disease need to be found.
CircRNAs can act as miRNA sponges to abolish the inhibitory effects of miRNAs on their target mRNAs, thus increasing the expression levels of mRNAs (14-16). In recent years, it has been confirmed that circRNAs play important roles in the pathogenesis of diseases and are potential molecular markers (12,13). Thus, it is extremely important to study the role of circRNAs to aid in the diagnosis and treatment of OLP. However, a review of the literature revealed that no studies of circRNAs in OLP appear to have been conducted to date.
The present study showed that there were large numbers of abnormally expressed circRNAs in OLP, including 83 upregulated and 52 downregulated circRNAs. The RT-qPCR results were consistent with the sequencing results (i.e., the results confirmed that the selected 10 circRNAs were abnormally expressed in OLP and provided further evidence of the sequencing data’s reliability). Among these 135 differentially expressed circRNAs, 19 were novel circRNAs. Of these 19, chr6:31238920-31324013- and chr19:4511523-4511918- were verified by RT-qPCR, and both were significantly upregulated in OLP. The host gene of chr6:31238920-31324013- was HLA-C, which plays an important role in the human immune system. The high cell-surface expression of HLA-C was related to aggravating cytotoxic T-cell responses (19). Hsa_circ_0006867 was downregulated in OLP and was derived from LRBA. LRBA participates in the regulation of the secretion and membrane deposition of immune effector molecules. A lack of LRBA leads to defects in regulatory T cells, which caused immune disorders (20). As is well known, OLP is a T-cell-mediated autoimmune disease (21,22). The abnormal expression of chr6:31238920-31324013- and hsa_circ_0006867 in OLP suggests that they may be involved in the T-cell-mediated immune process OLP. Also, this study showed that hsa_circ_0060927 was significantly upregulated. Its parental gene was CYP24A1, which encodes the protein CYP24A1. CYP24A1 is a mitochondrial enzyme, the main enzyme responsible for the degradation of active vitamin D, and has an important association with cancer (23,24). It has been reported that hsa_circ_0060927 is also abnormally expressed in uterine leiomyomas and colorectal cancer (25,26). The polymorphism of the CYP24A1 gene has been associated with the risk of OLP (27). Research has also shown that after CYP24A1 knockout, oral epithelial cells can regulate antibacterial activity by expressing higher levels of VDR-mediated (Vitamin D receptor mediated) genes (28). However, the question as to whether hsa_circ_0060927 plays a role in the occurrence of OLP needs to be addressed.
The GO enrichment analysis showed that the identified circRNAs were involved in 382 biological processes, 99 cellular components, and 54 molecular functions. According to fold enrichment ranking, 7 of the top 10 biological processes were related to immunity, such as “antigen processing and [the] presentation of endogenous peptide antigen[s],” the “positive regulation of macroautophagy,” and “antigen processing and [the] presentation of endogenous antigen[s].” Thus, it appears that circRNAs may be involved in the immunological process of OLP. The cell component item with the highest fold enrichment was the “MHC class I protein complex.” MHC I participates in recognition of T cells by binding to T-cell antigen receptors (29). Among 54 molecular function items, the most enriched was the “ubiquitin-like modifier activating enzyme activity.” Ubiquitin-like protein has been shown to play an important role in the process of protein folding (30). It is also involved in DNA damage responses, cell cycle control, cell signal transduction, protein transport, and an innate immune activation process (30,31). To date, no research related to ubiquitin in the field of OLP appears to have been conducted. The significant enrichment of the “ubiquitin-like modifier activating enzyme activity” suggests that it may play an important role in OLP. The GO function items enriched in this study indicate that circRNAs have various functions and may play a role in the occurrence of OLP.
The KEGG pathway analysis was performed to infer the function of circRNA by analyzing the pathway involving in circRNA-derived genes. Among 78 signal pathways that were enriched, the pathway with the highest enrichment score was the “natural killer cell-mediated cytotoxicity” pathway. Natural killer cells infiltrated the OLP tissues (32). CircRNAs may affect the occurrence of OLP by participating in the regulation of natural killer cell-mediated cytotoxicity. The enrichment scores ranked second to fourth were the “B-cell receptor signaling pathway,” the “cGMP-PKG signaling pathway,” and the “T-cell receptor signaling pathway.” These pathways all contained PI3K/AKT signals. The PI3K/AKT signal was abnormal in OLP, which participated in the immune regulation mechanism of OLP by regulating the crosstalk between the T cells and keratinocytes (33). CircRNAs may also participate in the immune regulation of OLP by regulating PI3K/AKT signaling. The “phospholipase D (PLD) signaling pathway” was enriched in both the upregulated and downregulated genes. Wang et al. (34) reported that the PLD2/MTOR/HIF-1α signal might play an important role in immune dysfunction in OLP patients. It has also been reported that PLD2 is highly expressed in OLP, and PLD2 promotes T-cell proliferation and pro-inflammatory phenotype differentiation in OLP (35).
Additionally, 6 immune-related signal pathways were enriched, which suggests that abnormally expressed circRNAs may be involved in regulating the immune process. We also enriched signal pathways related to viral infections and endocrine diseases and found that viral infection and endocrine factors may play a role in the occurrence of OLP. The pathways enriched by the KEGG analysis are of great significance to OLP’s occurrence and provide a theoretical basis for future research on the pathogenesis of OLP.
TargetScan and MiRanda software were used to predict the miRNA downstream of circRNAs. A ceRNA network diagram showed that abnormally expressed circRNAs in OLP might interact with a variety of miRNAs. Previous studies have shown that (36) that miR-27b-3p inhibited oral keratinocyte apoptosis by targeting CypD/Bcl-2 signaling in OLP, while miR-802 suppressed oral keratinocyte apoptosis by targeting Bcl-2 (37). We observed an interesting phenomenon, that is, that chr6:31238920-31324013- may interact with miR-27b-3p and miR-802 simultaneously. We also found that hsa_circ_0060927 may interact with miR-137. Notably, miR-137 is significantly downregulated in OLP, and an inverse correlation between miR-137 and CD8 has been found (38,39). Hsa_circ_0003943 may interact with miR-26b-5p (originally named miR-26b). MiR-26b in OLP has been reported to inhibit oral keratinocyte apoptosis by directly targeting PKCδ (40). Thus, circRNAs may adsorb downstream miRNAs and act as molecular sponges to regulate miRNAs, thereby changing mRNA expression level. Further research needs to be conducted to study the interactions between circRNAs and downstream target miRNAs and mRNAs in OLP.
Conclusions
In this study, an expression profile of abnormally expressed circRNAs in OLP was mapped for the first time. A total of 135 circRNAs were found to be differentially expressed between OLP and normal oral mucosa tissues. Of this 135 circRNAs, 19 were novel. The GO function and KEGG functional pathways analyses showed that the identified circRNAs might play important OLP roles. A circRNAs–microRNAs network was constructed to understand better the roles of circRNAs in the occurrence and development of OLP. Our study results may help other researchers elucidate the mechanism of OLP development and provide new clinical diagnostic markers and therapeutic targets.
Acknowledgments
We would like to thank Professor D.J. Cao and Professor Y.P. Pu for providing the normal oral mucosa tissues and CloudSeq Biotech (Shanghai, China) for providing the high-throughput sequencing service.
Funding: National Key R&D Program of China (81170968).
Footnote
Reporting Checklist: The authors have completed the MDAR reporting checklist. Available at http://dx.doi.org/10.21037/apm-20-2253
Data Sharing Statement: Available at http://dx.doi.org/10.21037/apm-20-2253
Peer Review File: Available at http://dx.doi.org/10.21037/apm-20-2253
Conflicts of Interest: All authors have completed the ICMJE uniform disclosure form (available at http://dx.doi.org/10.21037/apm-20-2253). The authors have no conflicts of interest to declare.
Ethical Statement: The authors are accountable for all aspects of the work in ensuring that questions related to the accuracy or integrity of any part of the work are appropriately investigated and resolved. The study was conducted in accordance with the Declaration of Helsinki (as revised in 2013). The study was approved by the Ethics Committee of the Ninth People’s Hospital of Shanghai Jiaotong University School of Medicine [No. 89 (2012) 21] and informed consent was taken from all the patients.
Open Access Statement: This is an Open Access article distributed in accordance with the Creative Commons Attribution-NonCommercial-NoDerivs 4.0 International License (CC BY-NC-ND 4.0), which permits the non-commercial replication and distribution of the article with the strict proviso that no changes or edits are made and the original work is properly cited (including links to both the formal publication through the relevant DOI and the license). See: https://creativecommons.org/licenses/by-nc-nd/4.0/.
References
- Shen ZY, Liu W, Zhu LK, et al. A retrospective clinico-pathological study on oral lichen planus and malignant transformation: analysis of 518 cases. Med Oral Patol Oral Cir Bucal 2012;17:e943-7. [Crossref] [PubMed]
- Parlatescu I, Tovaru M, Nicolae CL, et al. Oral health-related quality of life in different clinical forms of oral lichen planus. Clin Oral Investig 2020;24:301-8. [Crossref] [PubMed]
- Mozaffari HR, Sharifi R, Hayati M, et al. Evaluation of serum and salivary interferon-gamma levels in patients with oral lichen planus: a systematic review and meta-analysis of case-control studies. Oral Surg Oral Med Oral Pathol Oral Radiol 2019;127:210-7. [Crossref] [PubMed]
- Wang H, Zhang D, Han Q, et al. Role of distinct CD4(+) T helper subset in pathogenesis of oral lichen planus. J Oral Pathol Med 2016;45:385-93. [Crossref] [PubMed]
- Gonzalez-Moles MA, Scully C, Gil-Montoya JA. Oral lichen planus: controver-sies surrounding malignant transformation. Oral Dis 2008;14:229-43. [Crossref] [PubMed]
- Chen LL. The biogenesis and emerging roles of circular RNAs. Nat Rev Mol Cell Biol 2016;17:205-11. [Crossref] [PubMed]
- Li Y, Zheng Q, Bao C, et al. Circular RNA is enriched and stable in exosomes: a promising biomarker for cancer diagnosis. Cell Res 2015;25:981-4. [Crossref] [PubMed]
- Memczak S, Jens M, Elefsinioti A, et al. Circular RNAs are a large class of animal RNAs with regulatory potency. Nature 2013;495:333-8. [Crossref] [PubMed]
- Zhang Y, Zhang XO, Chen T, et al. Circular intron-ic long noncoding RNAs. Mol Cell 2013;51:792-806. [Crossref] [PubMed]
- Li Z, Huang C, Bao C, et al. Exon-intron circular RNAs regulate transcription in the nucleus. Nat Struct Mol Biol 2015;22:256-64. [Crossref] [PubMed]
- Hansen TB, Jensen TI, Clausen BH, et al. Natural RNA circles function as efficient microRNA sponges. Nature 2013;495:384-8. [Crossref] [PubMed]
- Li X, Yang L, Chen LL. The Biogenesis, Functions, and Challenges of Circular RNAs. Mol Cell 2018;71:428-42. [Crossref] [PubMed]
- Chen B, Huang S. Circular RNA: An emerging non-coding RNA as a regulator and biomarker in cancer. Cancer Lett 2018;418:41-50. [Crossref] [PubMed]
- Wang M, Yu F, Wu W, et al. Circular RNAs: A novel type of non-coding RNA and their potential implications in antiviral im-munity. Int J Biol Sci 2017;13:1497-506. [Crossref] [PubMed]
- Barrett SP, Salzman J. Circular RNAs: analysis, expression and potential functions. Development 2016;143:1838-47. [Crossref] [PubMed]
- Liang Y, Zhang Y, Xu L, et al. CircRNA Expression Pattern and ceRNA and miRNA-mRNA Networks Involved in Anther Development in the CMS Line of Brassica campestris. Int J Mol Sci 2019;20:4808. [Crossref] [PubMed]
- Yan L, Chen YG. Circular RNAs in Immune Response and Viral Infection. Trends Biochem Sci 2020;45:1022-34. [Crossref] [PubMed]
- Li Z, Cheng Y, Wu F, et al. The emerging landscape of circular RNAs in immunity: breakthroughs and challenges. Biomark Res 2020;8:25. [Crossref] [PubMed]
- van den Bogaard EH, Tijssen HJ, Rodijk-Olthuis D, et al. Cell Surface Expression of HLA-Cw6 by Human Epider-mal Keratinocytes: Positive Regulation by Cytokines, Lack of Correlation to a Vari-ant Upstream of HLA-C. J Invest Dermatol 2016;136:1903-6. [Crossref] [PubMed]
- Tesch VK, Abolhassani H, Shadur B, et al. Long-term outcome of LRBA deficiency in 76 patients after various treatment mo-dalities as evaluated by the immune deficiency and dysregulation activity (IDDA) score. J Allergy Clin Immunol 2020;145:1452-63. [Crossref] [PubMed]
- Chiang CP, Yu-Fong Chang J, Wang YP, et al. Oral lichen planus - Differential diagnoses, serum autoantibodies, hematinic deficiencies, and management. J Formos Med Assoc 2018;117:756-65. [Crossref] [PubMed]
- Carrozzo M, Porter S, Mercadante V, et al. Oral lichen planus: A disease or a spectrum of tissue reactions? Types, causes, diagnostic algorhythms, prognosis, management strategies. Periodontol 2000 2019;80:105-25. [Crossref] [PubMed]
- Osanai M, Lee GH. CYP24A1-induced vitamin D insufficiency promotes breast cancer growth. Oncol Rep 2016;36:2755-62. [Crossref] [PubMed]
- Shiratsuchi H, Wang Z, Chen G, et al. Oncogenic Poten-tial of CYP24A1 in Lung Adenocarcinoma. J Thorac Oncol 2017;12:269-80. [Crossref] [PubMed]
- Fazeli E, Piltan S, Sadeghi H, Gholami M, Azizi-Tabesh G, Yassaee F, et al. Ec-topic expression of CYP24A1 circular RNA hsa_circ_0060927 in uterine leiomyo-mas. J Clin Lab Anal 2020;34:e23114 [Crossref] [PubMed]
- Sadeghi H, Heiat M. Sadeghi H, Heiat M. A novel circular RNA hsa_circ_0060927 may serve as a potential diagnostic biomarker for human colorectal cancer. Mol Biol Rep 2020;47:6649-55. [Crossref] [PubMed]
- Kujundzic B, Zeljic K, Supic G, et al. Associa-tion of vdr, cyp27b1, cyp24a1 and mthfr gene polymorphisms with oral lichen planus risk. Clin Oral Investig 2016;20:781-9. [Crossref] [PubMed]
- Wang Q, Zhang W, Li H, et al. Effects of 25-hydroxyvitamin D3 on cathelicidin production and antibacterial function of human oral keratinocytes. Cell Immunol 2013;283:45-50. [Crossref] [PubMed]
- Rossjohn J, Gras S, Miles JJ, et al. T cell anti-gen receptor recognition of antigen-presenting molecules. Annu Rev Immunol 2015;33:169-200. [Crossref] [PubMed]
- Chanarat S, Mishra SK. Emerging Roles of Ubiquitin-like Proteins in Pre-mRNA Splicing. Trends Biochem Sci 2018;43:896-907. [Crossref] [PubMed]
- Fang Z, Pan Z. Essential Role of Ubiquitin-Fold Modifier 1 Conjugation in DNA Damage Response. DNA Cell Biol 2019;38:1030-9. [Crossref] [PubMed]
- Parolini S, Santoro A, Marcenaro E, et al. The role of chemerin in the colocalization of NK and dendritic cell subsets into inflamed tissues. Blood 2007;109:3625-32. [Crossref] [PubMed]
- Ma RJ, Tan YQ, Zhou G. Aberrant IGF1-PI3K/AKT/MTOR signaling pathway regulates the local immunity of oral lichen planus. Immunobiology 2019;224:455-61. [Crossref] [PubMed]
- Wang F, Zhang J, Zhou G. Deregulated phospholipase D2/mammalian target of rapamycin/hypoxia-inducible factor 1 alpha in peripheral T lymphocytes of oral li-chen planus correlated with disease severity. Arch Oral Biol 2019;98:26-31. [Crossref] [PubMed]
- Wang F, Zhang J, Zhou G. HIF1alpha/PLD2 axis linked to glycolysis induces T-cell immunity in oral lichen planus. Biochim Biophys Acta Gen Subj 2020;1864:129602 [Crossref] [PubMed]
- Chen J, Wang Y, Du G, et al. Down-regulation of miR-NA-27b-3p suppresses keratinocytes apoptosis in oral lichen planus. J Cell Mol Med 2019;23:4326-37. [Crossref] [PubMed]
- Zhao B, Xu N, Li R, et al. Vitamin D/VDR signaling suppresses microRNA-802-induced apoptosis of keratinocytes in oral lichen planus. FASEB J 2019;33:1042-50. [Crossref] [PubMed]
- Aghbari SMH, Gaafar SM, Shaker OG, et al. Evaluating the accuracy of microRNA27b and microRNA137 as biomarkers of activity and poten-tial malignant transformation in oral lichen planus patients. Arch Dermatol Res 2018;310:209-20. [Crossref] [PubMed]
- Aghbari SMH, Abushouk AI, Shakir OG, et al. Correlation be-tween tissue expression of microRNA-137 and CD8 in oral lichen planus. Clin Oral Investig 2018;22:1463-7. [Crossref] [PubMed]
- Du J, Gao R, Wang Y, et al. MicroRNA-26a/b have protective roles in oral lichen planus. Cell Death Dis 2020;11:15. [Crossref] [PubMed]