Anti-cytokines in the treatment of cancer cachexia
Introduction
Cancer-related cachexia (CRC) was recently defined by an international panel of experts as a “multifactorial syndrome characterized by an ongoing loss of skeletal muscle mass (with or without loss of fat mass) that cannot be fully reversed by conventional nutritional support and leads to progressive functional impairment” (1). It is recognized as a distinct entity from age-related loss of muscle mass, starvation, primary depression, malabsorption, and hyperthyroidism (2). Although the frequency of CRC may vary depending on the definition and criteria used for its diagnosis, it is considerably high (60–80%) (3-6), particularly among patients with lung, head and neck, upper gastrointestinal malignancies, and some aggressive forms of lymphomas (7,8). Important clinical findings of cachectic cancer patients include different degrees of weight loss, anorexia, fatigue, decreased muscle strength, reduced fat-free mass index, and biochemical disturbances such as anemia, low serum albumin, and high inflammatory markers (2). As compared to non-cachectic patients, patients suffering from CRC have lower levels of functionality, worse quality of life, more frequent treatment-related toxicity, and shorter overall survival (9-11). Furthermore, CRC is a source of psychological distress for both patients, and their caregivers (12-14).
Unfortunately, despite the clinical importance of this frequent and devastating syndrome, there are no broadly adopted guidelines to standardize its management (15). Moreover, there is a lack of effective pharmacological interventions to treat CRC, and current management mostly relies on non-pharmacological approaches such as nutrition education, and support, as well as exercise (16). Pharmacological agents that have been recommended as reasonable options in managing cancer cachexia are symptom-directed drugs like corticosteroids, and progesterone analogs (17-19). Although beneficial, the positive effects of these drugs are mainly on the anorectic component of the CRC syndrome, and their clinical use is limited by their side effect profile (19,20). Agents that have also been studied for the management of CRC include natural compounds (i.e., eicosapentanoic acid) (21,22), ghrelin analogs (i.e., anamorelin) (23,24), COX-2 inhibitors (i.e., celecoxib) (25,26), selective androgen receptor modulators (i.e., enobosarm) (27,28), synthetic cannabinoids (i.e., dronabinol) (29,30), anti-cytokines (i.e., thalidomide) (31,32), and others (33). In this article, we reviewed the current evidence on anti-cytokine interventions for the treatment of CRC as these constitute promising strategies to effectively manage this syndrome.
Pathogenesis of CRC and the role of cytokines
Although recent research has progressed in eliciting the underlying biological mechanisms driving the development of CRC, a complete understanding of its etiology and pathogenesis is yet to be achieved (34). Nevertheless, the hypercatabolic state triggering skeletal muscle and adipose tissue depletion in CRC is thought to be the consequence of a variable combination of different mechanisms, including alterations in energy balance with high levels of resting energy expenditure (REE) and reduced energy intake; hormonal and metabolic disturbances, both peripherally and in the central nervous system (CNS); and a pro-inflammatory/pro-cachectic environment induced by tumor byproducts and immune-system mediators (35). Among the several tumor and immune-system-derived inflammatory and pro-cachectic factors involved in the pathogenesis of CRC, cytokines such as IL-6, IL-1 beta, TNF-alpha, TWEAK (TNF-related weak inducer of apoptosis), and others, seem to play a central role in the development of CRC as they can determine proteolysis and lipolysis by a direct effect on target tissues, or indirectly by disrupting CNS controls of appetite and metabolic processes (35,36). Their central role on CRC pathogenesis has been suggested by studies conducted both in animal and in human subjects. In preclinical studies, for example, IL-1, TNF-alpha, and IL-6 have been linked to the development of weight loss, skeletal muscle catabolism, and adipose tissue depletion in rodents (37-39). On the other hand, blocking the same mediators with the administration of anti-cytokine antibodies was able to attenuate cachexia in animal tumor models (40,41). Also, gene knockout mice for the TNF-alpha receptor type I protein had less muscle wasting as compared to their wild-type counterparts in a fast-growing mouse tumor-induced cachexia model, highlighting the importance of TNF-alpha in the skeletal muscle depletion of CRC (42). Similarly, in clinical studies involving human subjects with different cancer types, high levels of peripheral inflammatory cytokines (i.e., IL-6, TNF-alpha) have been also associated with clinical and biochemical markers of cachexia like weight loss, low body mass index, high REE, and decreased levels of serum albumin, total protein, and hemoglobin (43-45). Taken together, these studies have supported the important role of inflammatory cytokines and pro-cachectic mediators in the pathogenesis of CRC, and, thus, have provided the rationale for the development of anti-cytokine agents to manage this common paraneoplastic syndrome. Figure 1 describes the core biological mechanisms of CRC pathogenesis, and the main targeted anti-cytokine treatments discussed in this review.
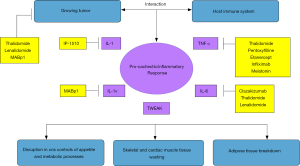
Anti-cytokine agents for CRC
Thalidomide
Thalidomide is a derivative of glutamic acid that has been shown to possess anti-inflammatory, immunomodulatory, anti-angiogenic, sedative and anti-emetic effects (46,47). Although its mechanism of action is not completely understood, it seems to involve the suppression of several cytokines (i.e., TNF-alpha, IL-6), and angiogenesis mediators (i.e., VEGF, FGF), as well as the inhibition of NF-kappa B and downregulation of COX-2 (48). Because of these properties, thalidomide has been studied in the management of auto-immune conditions like Bechet’s disease, and rheumatoid arthritis (49), as an anti-neoplastic therapy in different tumor types (49,50), and in the treatment of cachexia, and its related symptoms caused by malignant and non-malignant diseases such as the HIV-associated wasting syndrome (51,52). In the treatment of CRC, several clinical trials have studied the use of thalidomide in doses ranging from 100 to 200 mg/day, as a single agent or in combination with other interventions to manage cachexia in different cancer populations (31,53-60). At least five of the CRC trials evaluating thalidomide were randomized controlled (Table 1) (31,55,56,58-60). In the first of these, Gordon et al. compared thalidomide 200 mg daily for 24 weeks with placebo in a population of inoperable pancreatic cancer patients with more than 10% of weight loss in the last 6 months, and a life expectancy ≥6 weeks (31). Fifty patients were randomized to both arms, and 33 were evaluable (pre-planned evaluable sample size was 34 for the primary endpoint analysis at week 4). While patients in the placebo group lost an average of 2.21 kg of body weight (primary endpoint), and 4.6 cm3 of bone free muscle mass as measured by dual-energy X-ray absorptiometry (DEXA), patients receiving thalidomide gained 0.37 kg [absolute difference =−2.59 kg, (95% CI, −4.3 to −0.8), P=0.005], and 1.0 cm3 [absolute difference =−5.6 cm3 (95% CI, −8.9 to −2.2, P=0.002] in weight, and arm muscle mass, respectively. Thalidomide was overall well tolerated (31). In a similar study design, Wilkes et al. have also tested thalidomide (200 mg/day) against placebo, but using a slightly different drug exposure period (6 weeks), and in a different population of incurable esophageal cancer patients with life expectancy of more than 8 weeks (56). No specific CRC diagnostic criteria were employed for patient inclusion. Thirty-four patients were randomized to the two study groups. However, because of a high attrition rate due to disease progression, elective withdrawal, and drug toxicity, only 8 patients were evaluable in the thalidomide group at week 6, compromising the study power to detect outcomes differences between arms. No statistically significant differences between study groups were found in any of the primary [eight change, and lean body mass (LBM) by DEXA] or secondary endpoints (REE by indirect calorimetry, other body composition measures, Karnofsky Index, fatigue by Piper questionnaire, serum levels of cytokines, and survival). Nine of the 17 patients on thalidomide arm had drug-related adverse events, mainly rash, and hypersomnolence (56). More recently, in another placebo-controlled randomized trial designed to evaluate the effects of thalidomide (100 mg/day) on anorexia-cachexia-related symptoms, body composition, resting metabolic rate, and serum cytokines in a population of advanced cancer patients with various tumor types, Yennurajalingam et al. have also had issues with poor accrual and high attrition rates that probably influenced the study outcomes (58). From the 31 patients that entered the study, a third dropped out before the study’s primary endpoint analysis at day 15, mainly because of hospitalization due to disease progression, and non-adherence. The authors found no between-group difference in the outcomes evaluated. Toxicity was minimal and none of the patients withdrew because of thalidomide-related adverse events (61). In contrary to the limited results attained by small randomized trials that investigated single-agent thalidomide, many of which were also underpowered due to high attrition rates, studies evaluating thalidomide in combination with other interventions, and in larger cohorts had more encouraging results (55,59). In a 5-arm single-center trial, Mantovani et al. randomized 332 advanced cancer patients with a ≥5% loss of ideal or pre-illness weight within last 3 months, and life expectancy of ≥4 months, to receive one of the following interventions: (I) medroxyprogesterone 500 mg/day or megestrol acetate 320 mg/day; (II) 2.2 g/day of oral supplementation with eicosapentaenoic acid; (III) L-carnitine 4 g/day; (IV) thalidomide 200 mg/day; and (V) a combination of all interventions. Final analysis at week 16 comparing only arms 3, 4, and 5 (arms 1, and 2 were withdrawn because they fulfilled inferiority rules in the pre-planned interim analysis), showed that the combined intervention was significantly superior to other arms for the primary outcomes of LBM by DEXA (P=0.007), REE by indirect calorimetry (P=0.028), and fatigue (P=0.035) as measured by the Multidimensional Fatigue Symptom Inventory-Short Form. All patients in this study were evaluable (55). Finally, in a more recent trial using the same CRC, and prognostic criteria as the prior 5-arm study, Wen et al. randomized 108 advanced cancer patients to megestrol acetate (320 mg/day) alone or in combination with thalidomide (100 mg/day) for 8 weeks. The combination arm had significant improvements in primary endpoints of body weight (P=0.025), fatigue (P<0.01), and quality of life (P=0.01) as compared to single agent megestrol. In addition, combination therapy group had also better ECOG-PS (P=0.02), and grip strength (P=0.05), as well as lower Glasgow Prognostic Score (P=0.02), and lower levels of TNF-alpha (P=0.01), and IL-6 (P<0.01) (59). The mixed results achieved by these randomized clinical trials have prevented thalidomide to be recommended as a pharmacological therapy in the management of CRC in clinical practice. Nevertheless, because of its broad mechanisms of action targeting multiple pathways involved in CRC pathogenesis, overall good tolerability when used in doses below 200 mg/day, and its low cost, at least in developing countries (62), it still remains an attractive agent that warrants further research as a CRC treatment option. Lenalidomide, a derivative of thalidomide with similar properties of the parent compound, is also being investigated for the treatment of CRC in a randomized-controlled clinical trial. Results of this study are not yet available (63).
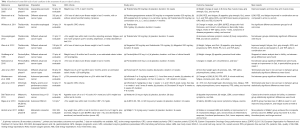
Full table
Pentoxifylline, etanercept, infliximab, and melatonin
TNF-alpha has been suggested to be one of the key pro-inflammatory mediators involved in the pathogenesis of CRC (33,36). This has been supported by both preclinical and clinical data, and has provided the conceptual basis for the development of anti-TNF strategies to treat the CRC syndrome (38,41,42,44). Pentoxifylline, a blood viscosity reducer agent; the monoclonal antibodies etanercept, and infliximab; and melatonin, a pleotropic hormone used to treat sleep disturbances, have been investigated in clinical trials as anti-TNF therapies for managing the CRC syndrome (32,64-67). The methylxanthine derivative pentoxifylline was the first drug within this group to be tested in CRC randomized-controlled trials. Unfortunately, these studies failed to demonstrate any beneficial effect of pentoxifylline to advanced cancer patients with cachexia. In a multicenter study conducted by the North Central Cancer Treatment Group, pentoxifylline (400 mg, three times a day) was not better than the identical placebo in improving body weight, appetite, or perceived food intake in a population of 70 advanced cancer patients with ≥5% loss of ideal or pre-illness weight in the previous 2 months from study entry. The trial was closed earlier due to futility (32). Negative results with pentoxifylline for managing CRC have also been observed in a recent randomized placebo-controlled trial conducted in a cohort of cachectic advanced cancer patients in Iran. After 8 weeks of follow-up, no statistically significant difference were found between pentoxifylline and placebo groups in study outcomes except for a better quality of life as measured by the Short Form 36 survey (SF36) in the anti-TNF arm at week 4 that was not anymore found in the 8-week analysis (64). Similarly to what have been observed in the pentoxifylline clinical trials, both the monoclonal antibodies etanercept, and infliximab have shown no benefit in treating cancer cachexia (65-67). In a proof-of-concept multicenter clinical trial, Jatoi et al. randomized 64 advanced cancer patients expected to live 3 or more months to receive the dimeric fusion protein etanercept (25 mg/day subcutaneous twice weekly) during 24 weeks or placebo during the same period. The trial was terminated earlier due to low accrual, and no significant differences were observed between etanercept and placebo in non-fluid weight gain, patient-reported symptoms, quality of life or survival (65). The same group of researchers also tested the anti-TNF monoclonal antibody infliximab to treat cachexia in a population of 64 elderly, and/or poor performance status non-small cell lung cancer (NSCLC) patients. The cohort was randomized to receive infliximab (5 mg/kg/day on day 1, weeks 1, 3, and 5 in the first 8-week cycle, then on day 1, weeks 1 and 5 every 8-week cycle) for 4–6 months or placebo. All patients received docetaxel (36 mg/m2 intravenously, 6 weeks on, 2 weeks off) during the same period. This trial was also stopped earlier due to low accrual. As compared to placebo, patients in the intervention group had more fatigue, and worse functional and physical well-being. No benefits were found with infliximab therapy (66). Another randomized controlled trial comparing infliximab plus gemcitabine versus gemcitabine plus placebo in cachectic patients with stage II–IV pancreatic cancer have also had negative results (67). Finally, melatonin has been evaluated in the treatment of CRC. Although non-blinded and/or non-placebo controlled studies were able to demonstrate positive effects of the use of melatonin as anti-CRC agent, this was not confirmed in a recent randomized, double-blind, placebo-controlled trial that investigated the use of melatonin (20 mg at night) for managing anorexia and other CRC-related symptoms in a population of advanced lung or gastrointestinal cancer patients. The study was closed at the interim analysis for futility (68). Despite that none of these anti-TNF agents were beneficial in CRC clinical trials, it is not possible to reject the prior evidence pointing to the central role of TNF-alpha in CRC pathogeneses, as issues such as low accrual, sample size, and the use of drugs that interfere with only one of the multiple cytokines involved in the development of CRC may have prevented these studies to have positive results.
MABp1
IL-1 alpha has been shown to be an early mediator of the inflammatory response, triggering the synthesis of other cytokines, and driving both tumor growth and cachexia (35,37,69-71). Thus, it has also been identified as an attractive therapeutic target in the management of the CRC syndrome. MABp1, a natural IgG1k human monoclonal antibody targeting IL-1 alpha has been recently evaluated as a new pharmacological treatment of CRC and its related symptoms (72,73). Interesting findings were first demonstrated in an open-label, phase I, dose-escalation trial to evaluate MABp1 safety, and tolerability. In this study, 52 metastatic cancer patients were exposed, every 3 weeks, to four dose levels of intravenous MABp1 (0.25, 0.75, 1.25, and 3.75 mg/kg). Therapy was overall well tolerated with proteinuria, nausea, and fatigue being the most frequent possibly drug-related side effects. In addition, as compared to baseline, patients completing the 8-week secondary outcomes assessments had significant improvements in mean LBM as assessed by DEXA (P=0.02), fatigue (P=0.008), appetite loss (P=0.02), and pain (P=0.02) symptom scores, as well as social (P=0.04), emotional (P=0.03), role function (P=0.006), and quality of life (P=0.02) scores as measured by the European Organization for Research and Treatment of Cancer Quality of Life Questionnaire (EORTC-QLQ C30). From 34 patients evaluable for tumor response, 10 had stable disease and one had a partial response (72). More recently, a larger, multicenter, double-blind, placebo-controlled phase III trial evaluated the effects of MABp1 on a composite primary endpoint that included patient-reported (EORTC-QLQ-C30), and body composition assessments (LBM by DEXA). Three hundred and thirty three advanced colorectal cancer patients with any involuntary weight loss ≤20% within last 6 months or ≥10 pg/mL serum IL-6 and who also had disease-related symptoms (>10 points in EORTC-QLQ-C30 anorexia, fatigue or pain symptom scores), and impaired quality of life (<90 points in EORTC-QLQ-C30 role, emotional, and social function scores) were randomly allocated to receive MABp1 (7.5 mg/kg IV) every 2 weeks for 8 weeks or placebo. As compared to placebo, a higher proportion of patients in the Mabp1 group achieved the combined primary endpoint (33% vs. 19%, P=0.004) at week 8. Also, exploratory secondary outcomes analysis showed that patients in the intervention arm had significantly lower levels of serum IL-6 (1.6 vs. 9.9 pg/mL, P=0.01), and thrombocytosis (14×109vs. 40×109/L, P=0.005) as well as longer median survival (6.1 vs. 2.4 months, P=0.0002). There were no significant differences in the rate of adverse events between groups. The most common grade 3 adverse events in MABp1 group were anemia (4%), fatigue (3%), peripheral edema (2%), and abdominal pain (2%) (73). Despite these promising results, more research is needed to establish a role for this agent in the management of CRC.
Clazakizumab
The observation that IL-6 inhibition is able to detain the development of cachectic features in preclinical animal studies has raised the interest in targeting this pro-inflammatory/pro-cachectic factor also in the clinical setting (40,74). Although several anti-IL-6 drugs are currently under development, the humanized monoclonal antibody clazakizumab, previously ALD518 or BMS-945429, is the only specific anti-IL6 agent that has been evaluated in clinical trials for the treatment of CRC (75-78). After a phase I study demonstrating the safety of clazakizumab in a small cohort of 9 advanced cancer patients, a phase II study was conducted in a population of 124 incurable NSCLC patients diagnosed with cachexia defined by >5% loss of body weight within last 3 months (76-78). Patients were randomly assigned to receive one of three doses of intravenous clazakizumab (80, 160, or 320 mg) every 8 weeks for 24 weeks or placebo. Only 29 patients were able to complete all the pre-planned study evaluations during the 6-month period. The majority either died due progressive disease (n=52) or failed to attend all the scheduled study visits (n=38). A preliminary pooled analysis including patients receiving any of the three clazakizumab doses revealed that, as compared to placebo, the intervention group had a lower proportion of patients losing more than 5% of LBM as measured by DEXA (3% vs. 20%, P=0.05) at week 12, and reported better lung symptoms score (15.1 vs. 14.1, P<0.0006) and fatigue score (22.9 vs. 21.3, P=0.025) at week 2 as assessed by the Functional Assessment of Cancer Therapy—Lung Cancer Subscale, and the Functional Assessment of Chronic Illness Therapy-Fatigue, respectively. One patient had a serious adverse event (rectal hemorrhage) considered possibly related to clazakizumab. No significant differences between clazakizumab and placebo study groups were observed in the rate of serious adverse events. The most common adverse effects in the intervention group were dyspnea (18%), chest pain (11%), and hemoptysis (11%) (76,78). Further research on this and other anti-IL-6 CRC agents are warranted.
Other anti-cytokine agents
Other two anti-cytokine drugs, IP-1510, a synthetic IL-1 receptor antagonist, and OHR/AVR118, a peptide-nucleic acid that has immunomodulatory, and anti-inflammatory properties by acting on different cytokines, have been investigated for the treatment of CRC (79,80). Preliminary results of a small phase I/II clinical trial to evaluate safety and toxicity of the administration of IP-1510 (1 mg subcutaneous twice a day for 28 days) given to a population of advanced cancer patients were presented at the 6th Cachexia Conference in Milan, Italy. No specific CRC diagnostic criteria were reported for patient inclusion. The majority (evaluable =20/29) were able to complete the scheduled treatment, and the drug was deemed to be well tolerated with no serious adverse events being described. Additionally, 17 patients gain or stabilized their weight, and significant improvements were noticed in the Karnofsky performance status (P≤0.01), and in the Edmonton Symptom Assessment Scale (ESAS) appetite (P≤0.01), and depression scores (P≤0.01) (81,82).
With regards to the use of the peptide-nucleic acid OHR/AVR118 to manage CRC and its related symptoms, the most recent clinical data was also presented in an international cachexia conference (83). In this phase I/II study conducted in a population of stage III–IV cancer patients, Chasen et al. investigated the effects of OHR/AVR 118 (4 mL subcutaneous once a day) for 28 days on appetite, early satiety and nutritional intake as measured by the ESAS, Dyspepsia Symptom Severity Index, and the patient-generated subjective global assessment. As compared to baseline, evaluable patients completing study assessments (n=18) had significant improvements in appetite (P=0.001), and nutritional status scores (P=0.025). At the end of the treatment period, body weight, fat, and muscle mass was also stabilized. The authors reported that drug tolerability was good (83). Final results of studies evaluating these two agents are still pending publication.
Conclusions and future perspectives
Although preclinical and clinical research studies have made progress in understanding the pathogenesis of CRC, and in identifying biological pathways that could possibly be targeted with pharmacological therapies, there are still no effective drugs approved for the treatment of CRC and its related symptoms, and it remains an unmet clinical problem. The use of agents targeting cytokines involved in the pathogenesis of CRC has emerged as an attractive strategy to manage the syndrome. However, the majority of clinical trials evaluating anti-cytokines CRC therapies were not able to achieve positive outcomes. This can be explained by several reasons. Until recently, there were no consensus-based CRC definition, diagnostic criteria, or classification system developed specifically to reflect the particular characteristics of cachexia of the adult cancer population, which have resulted in the inclusion of very heterogeneous patient populations in CRC clinical trials. Also, most studies recruited patients at or approaching the late disease stages, with short life expectancy, when cachexia might be already refractory to clinical interventions, and when the possibility of attrition due to disease progression or death is very high. Moreover, many anti-cytokines tested have no anti-cancer effect and have a very limited mechanism of action, in which only one or few pro-cachectic mediators are targeted. Interestingly, thalidomide (either alone or in combined interventions) and MABp1, drugs that have shown to interfere with multiple CRC cytokines and pathways, while also possessing anti-cancer properties, were the anti-cytokines agents that have demonstrated the most prominent clinical benefits in larger phase II or III clinical trials, confirming the need of using a multitarget pharmacological therapy to address CRC complex underlying mechanisms. Therefore, based on the evidence built until now, anti-cytokines agents continue to be a promising CRC therapy. To achieve better outcomes and overcome methodological challenges, the design of future CRC clinical trials involving anti-cytokines will need to include standardized inclusion criteria, valid, and clinically meaningful outcome measures, and the use of low toxicity therapies targeting multiple and redundant pro-cachectic molecules and pathways in earlier phases of the cancer cachexia continuum. This might make possible that an anti-cytokine is added to a multimodal approach to manage CRC multidimensional syndrome.
Acknowledgements
The authors would like to thank Dr. Eduardo Bruera and Dr. Sriram Yennurajalingam for their guidance on the current work.
Footnote
Conflicts of Interest: The authors have no conflicts of interest to declare.
References
- Fearon K, Strasser F, Anker SD, et al. Definition and classification of cancer cachexia: an international consensus. Lancet Oncol 2011;12:489-95. [Crossref] [PubMed]
- Evans WJ, Morley JE, Argiles J, et al. Cachexia: a new definition. Clin Nutr 2008;27:793-9. [Crossref] [PubMed]
- von Haehling S, Anker SD. Cachexia as a major underestimated and unmet medical need: facts and numbers. J Cachexia Sarcopenia Muscle 2010;1:1-5. [Crossref] [PubMed]
- Lees J. Incidence of weight loss in head and neck cancer patients on commencing radiotherapy treatment at a regional oncology centre. Eur J Cancer Care (Engl) 1999;8:133-6. [Crossref] [PubMed]
- Dewys WD, Begg C, Lavin PT, et al. Prognostic effect of weight loss prior to chemotherapy in cancer patients. Eastern Cooperative Oncology Group. Am J Med 1980;69:491-7. [Crossref] [PubMed]
- Bachmann J, Heiligensetzer M, Krakowski-Roosen H, et al. Cachexia worsens prognosis in patients with resectable pancreatic cancer. J Gastrointest Surg 2008;12:1193-201. [Crossref] [PubMed]
- Laviano A, Meguid MM. Nutritional issues in cancer management. Nutrition 1996;12:358-71. [Crossref] [PubMed]
- Laviano A, Meguid MM, Inui A, et al. Therapy insight: Cancer anorexia-cachexia syndrome--when all you can eat is yourself. Nat Clin Pract Oncol 2005;2:158-65. [Crossref] [PubMed]
- Capuano G, Gentile PC, Bianciardi F, et al. Prevalence and influence of malnutrition on quality of life and performance status in patients with locally advanced head and neck cancer before treatment. Support Care Cancer 2010;18:433-7. [Crossref] [PubMed]
- Ross PJ, Ashley S, Norton A, et al. Do patients with weight loss have a worse outcome when undergoing chemotherapy for lung cancers? Br J Cancer 2004;90:1905-11. [Crossref] [PubMed]
- Hendifar AE, Chang JI, Huang BZ, et al. Cachexia, and not obesity, prior to pancreatic cancer diagnosis worsens survival and is negated by chemotherapy. J Gastrointest Oncol 2018;9:17-23. [Crossref] [PubMed]
- Rhondali W, Chisholm GB, Daneshmand M, et al. Association between body image dissatisfaction and weight loss among patients with advanced cancer and their caregivers: a preliminary report. J Pain Symptom Manage 2013;45:1039-49. [Crossref] [PubMed]
- Oberholzer R, Hopkinson JB, Baumann K, et al. Psychosocial effects of cancer cachexia: a systematic literature search and qualitative analysis. J Pain Symptom Manage 2013;46:77-95. [Crossref] [PubMed]
- Cooper C, Burden ST, Cheng H, et al. Understanding and managing cancer-related weight loss and anorexia: insights from a systematic review of qualitative research. J Cachexia Sarcopenia Muscle 2015;6:99-111. [Crossref] [PubMed]
- Dev R, Wong A, Hui D, et al. The Evolving Approach to Management of Cancer Cachexia. Oncology (Williston Park) 2017;31:23-32. [PubMed]
- NCCN Clinical Practice Guidelines in Oncology. Palliative Care Version I. 2018. Available online: https://www.nccn.org/professionals/physician_gls/default.aspx#palliative
- Miller S, McNutt L, McCann MA, et al. Use of corticosteroids for anorexia in palliative medicine: a systematic review. J Palliat Med 2014;17:482-5. [Crossref] [PubMed]
- Moertel CG, Schutt AJ, Reitemeier RJ, et al. Corticosteroid therapy of preterminal gastrointestinal cancer. Cancer 1974;33:1607-9. [Crossref] [PubMed]
- Ruiz Garcia V, López-Briz E, Carbonell Sanchis R, et al. Megestrol acetate for treatment of anorexia-cachexia syndrome. Cochrane Database Syst Rev 2013;3:Cd004310. [PubMed]
- Dev R, Del Fabbro E, Bruera E. Association between megestrol acetate treatment and symptomatic adrenal insufficiency with hypogonadism in male patients with cancer. Cancer 2007;110:1173-7. [Crossref] [PubMed]
- Fearon KC, Barber MD, Moses AG, et al. Double-Blind, Placebo-Controlled, Randomized Study of Eicosapentaenoic Acid Diester in Patients With Cancer Cachexia. J Clin Oncol 2006;24:3401-7. [Crossref] [PubMed]
- Dewey A, Baughan C, Dean T, et al. Eicosapentaenoic acid (EPA, an omega-3 fatty acid from fish oils) for the treatment of cancer cachexia. Cochrane Database Syst Rev 2007;1:Cd004597. [PubMed]
- Takayama K, Katakami N, Yokoyama T, et al. Anamorelin (ONO-7643) in Japanese patients with non-small cell lung cancer and cachexia: results of a randomized phase 2 trial. Support Care Cancer 2016;24:3495-505. [Crossref] [PubMed]
- Garcia JM, Boccia RV, Graham CD, et al. Anamorelin for patients with cancer cachexia: an integrated analysis of two phase 2, randomised, placebo-controlled, double-blind trials. Lancet Oncol 2015;16:108-16. [Crossref] [PubMed]
- Lai V, George J, Richey L, et al. Results of a pilot study of the effects of celecoxib on cancer cachexia in patients with cancer of the head, neck, and gastrointestinal tract. Head Neck 2008;30:67-74. [Crossref] [PubMed]
- Mantovani G, Maccio A, Madeddu C, et al. Phase II nonrandomized study of the efficacy and safety of COX-2 inhibitor celecoxib on patients with cancer cachexia. J Mol Med (Berl) 2010;88:85-92. [Crossref] [PubMed]
- Dobs AS, Boccia RV, Croot CC, et al. Effects of enobosarm on muscle wasting and physical function in patients with cancer: a double-blind, randomised controlled phase 2 trial. Lancet Oncol 2013;14:335-45. [Crossref] [PubMed]
- Crawford J, Prado CM, Johnston MA, et al. Study Design and Rationale for the Phase 3 Clinical Development Program of Enobosarm, a Selective Androgen Receptor Modulator, for the Prevention and Treatment of Muscle Wasting in Cancer Patients (POWER Trials). Curr Oncol Rep 2016;18:37. [Crossref] [PubMed]
- Jatoi A, Windschitl HE, Loprinzi CL, et al. Dronabinol versus megestrol acetate versus combination therapy for cancer-associated anorexia: a North Central Cancer Treatment Group study. J Clin Oncol 2002;20:567-73. [Crossref] [PubMed]
- Strasser F, Luftner D, Possinger K, et al. Comparison of orally administered cannabis extract and delta-9-tetrahydrocannabinol in treating patients with cancer-related anorexia-cachexia syndrome: a multicenter, phase III, randomized, double-blind, placebo-controlled clinical trial from the Cannabis-In-Cachexia-Study-Group. J Clin Oncol 2006;24:3394-400. [Crossref] [PubMed]
- Gordon JN, Trebble TM, Ellis RD, et al. Thalidomide in the treatment of cancer cachexia: a randomised placebo controlled trial. Gut 2005;54:540-5. [Crossref] [PubMed]
- Goldberg RM, Loprinzi CL, Mailliard JA, et al. Pentoxifylline for treatment of cancer anorexia and cachexia? A randomized, double-blind, placebo-controlled trial. J Clin Oncol 1995;13:2856-9. [Crossref] [PubMed]
- Aoyagi T, Terracina KP, Raza A, et al. Cancer cachexia, mechanism and treatment. World J Gastrointest Oncol 2015;7:17-29. [Crossref] [PubMed]
- Mondello P, Mian M, Aloisi C, et al. Cancer cachexia syndrome: pathogenesis, diagnosis, and new therapeutic options. Nutr Cancer 2015;67:12-26. [Crossref] [PubMed]
- Baracos VE, Martin L, Korc M, et al. Cancer-associated cachexia. Nat Rev Dis Primers 2018;4:17105. [Crossref] [PubMed]
- Argiles JM, Busquets S, Toledo M, et al. The role of cytokines in cancer cachexia. Curr Opin Support Palliat Care 2009;3:263-8. [Crossref] [PubMed]
- Fong Y, Moldawer LL, Marano M, et al. Cachectin/TNF or IL-1 alpha induces cachexia with redistribution of body proteins. Am J Physiol 1989;256:R659-65. [PubMed]
- Ling PR, Schwartz JH, Bistrian BR. Mechanisms of host wasting induced by administration of cytokines in rats. Am J Physiol 1997;272:E333-9. [PubMed]
- Chen JL, Walton KL, Qian H, et al. Differential Effects of IL6 and Activin A in the Development of Cancer-Associated Cachexia. Cancer Res 2016;76:5372-82. [Crossref] [PubMed]
- Zaki MH, Nemeth JA, Trikha M. CNTO 328, a monoclonal antibody to IL-6, inhibits human tumor-induced cachexia in nude mice. Int J Cancer 2004;111:592-5. [Crossref] [PubMed]
- Gelin J, Moldawer LL, Lonnroth C, et al. Role of endogenous tumor necrosis factor alpha and interleukin 1 for experimental tumor growth and the development of cancer cachexia. Cancer Res 1991;51:415-21. [PubMed]
- Llovera M, Garcia-Martinez C, Lopez-Soriano J, et al. Role of TNF receptor 1 in protein turnover during cancer cachexia using gene knockout mice. Mol Cell Endocrinol 1998;142:183-9. [Crossref] [PubMed]
- Kuroda K, Nakashima J, Kanao K, et al. Interleukin 6 is associated with cachexia in patients with prostate cancer. Urology 2007;69:113-7. [Crossref] [PubMed]
- Staal-van den Brekel AJ, Dentener MA, Schols AM, et al. Increased resting energy expenditure and weight loss are related to a systemic inflammatory response in lung cancer patients. J Clin Oncol 1995;13:2600-5. [Crossref] [PubMed]
- Wu J, Huang C, Xiao H, et al. Weight loss and resting energy expenditure in male patients with newly diagnosed esophageal cancer. Nutrition 2013;29:1310-4. [Crossref] [PubMed]
- Gordon JN, Goggin PM. Thalidomide and its derivatives: emerging from the wilderness. Postgrad Med J 2003;79:127-32. [Crossref] [PubMed]
- Peuckmann V, Fisch M, Bruera E. Potential novel uses of thalidomide: focus on palliative care. Drugs 2000;60:273-92. [Crossref] [PubMed]
- Franks ME, Macpherson GR, Figg WD. Thalidomide. Lancet 2004;363:1802-11. [Crossref] [PubMed]
- Mujagic H, Chabner BA, Mujagic Z. Mechanisms of action and potential therapeutic uses of thalidomide. Croat Med J 2002;43:274-85. [PubMed]
- Eisen T, Boshoff C, Mak I, et al. Continuous low dose Thalidomide: a phase II study in advanced melanoma, renal cell, ovarian and breast cancer. Br J Cancer 2000;82:812-7. [Crossref] [PubMed]
- Reyes-Teran G, Sierra-Madero JG, Martinez del Cerro V, et al. Effects of thalidomide on HIV-associated wasting syndrome: a randomized, double-blind, placebo-controlled clinical trial. Aids 1996;10:1501-7. [Crossref] [PubMed]
- Reid J, Mills M, Cantwell M, et al. Thalidomide for managing cancer cachexia. Cochrane Database Syst Rev 2012;4:Cd008664. [PubMed]
- Bruera E, Neumann CM, Pituskin E, et al. Thalidomide in patients with cachexia due to terminal cancer: preliminary report. Ann Oncol 1999;10:857-9. [Crossref] [PubMed]
- Khan ZH, Simpson EJ, Cole AT, et al. Oesophageal cancer and cachexia: the effect of short-term treatment with thalidomide on weight loss and lean body mass. Aliment Pharmacol Ther 2003;17:677-82. [Crossref] [PubMed]
- Mantovani G, Maccio A, Madeddu C, et al. Randomized phase III clinical trial of five different arms of treatment in 332 patients with cancer cachexia. Oncologist 2010;15:200-11. [Crossref] [PubMed]
- Wilkes EA, Selby AL, Cole AT, et al. Poor tolerability of thalidomide in end-stage oesophageal cancer. Eur J Cancer Care (Engl) 2011;20:593-600. [Crossref] [PubMed]
- Davis M, Lasheen W, Walsh D, et al. A Phase II dose titration study of thalidomide for cancer-associated anorexia. J Pain Symptom Manage 2012;43:78-86. [Crossref] [PubMed]
- Yennurajalingam S, Willey JS, Palmer JL, et al. The role of thalidomide and placebo for the treatment of cancer-related anorexia-cachexia symptoms: results of a double-blind placebo-controlled randomized study. J Palliat Med 2012;15:1059-64. [Crossref] [PubMed]
- Wen HS, Li X, Cao YZ, et al. Clinical studies on the treatment of cancer cachexia with megestrol acetate plus thalidomide. Chemotherapy 2012;58:461-7. [Crossref] [PubMed]
- Xie M, Chen X, Qin S, et al. Clinical study on thalidomide combined with cinobufagin to treat lung cancer cachexia. J Cancer Res Ther 2018;14:226-32. [Crossref] [PubMed]
- Yennurajalingam S, Bruera E. Role of corticosteroids for fatigue in advanced incurable cancer: is it a 'wonder drug' or 'deal with the devil'. Curr Opin Support Palliat Care 2014;8:346-51. [Crossref] [PubMed]
- Paumgartten FJ. Thalidomide and its analogues: comparative clinical efficacy and safety, and cost-effectiveness. Cadernos de saude publica 2014;30:684-6. [Crossref] [PubMed]
- Strasser F, Oberholzer R, Blum D, et al. Lenalidomide in solid tumor patients with inflammatory cancer cachexia: A multicenter, randomized, double-blind, proof-of-concept study of fixed dose or CRP-response-guided dose or placebo. J Clin Oncol 2011;29:TPS239. [Crossref]
- Mehrzad V, Afshar R, Akbari M. Pentoxifylline treatment in patients with cancer cachexia: A double-blind, randomized, placebo-controlled clinical trial. Adv Biomed Res 2016;5:60. [Crossref] [PubMed]
- Jatoi A, Dakhil SR, Nguyen PL, et al. A placebo‐controlled double blind trial of etanercept for the cancer anorexia/weight loss syndrome. Cancer 2007;110:1396-403. [Crossref] [PubMed]
- Jatoi A, Ritter HL, Dueck A, et al. A placebo-controlled, double-blind trial of infliximab for cancer-associated weight loss in elderly and/or poor performance non-small cell lung cancer patients (N01C9). Lung Cancer 2010;68:234-9. [Crossref] [PubMed]
- Wiedenmann B, Malfertheiner P, Friess H, et al. A multicenter, phase II study of infliximab plus gemcitabine in pancreatic cancer cachexia. J Support Oncol 2008;6:18-25. [PubMed]
- Del Fabbro E, Dev R, Hui D, et al. Effects of melatonin on appetite and other symptoms in patients with advanced cancer and cachexia: a double-blind placebo-controlled trial. J Clin Oncol 2013;31:1271-6. [Crossref] [PubMed]
- Kumar S, Kishimoto H, Chua HL, et al. Interleukin-1 alpha promotes tumor growth and cachexia in MCF-7 xenograft model of breast cancer. Am J Pathol 2003;163:2531-41. [Crossref] [PubMed]
- Voronov E, Shouval DS, Krelin Y, et al. IL-1 is required for tumor invasiveness and angiogenesis. Proc Natl Acad Sci U S A 2003;100:2645-50. [Crossref] [PubMed]
- Dinarello CA, Simon A, van der Meer JW. Treating inflammation by blocking interleukin-1 in a broad spectrum of diseases. Nat Rev Drug Discov 2012;11:633-52. [Crossref] [PubMed]
- Hong DS, Hui D, Bruera E, et al. MABp1, a first-in-class true human antibody targeting interleukin-1alpha in refractory cancers: an open-label, phase 1 dose-escalation and expansion study. Lancet Oncol 2014;15:656-66. [Crossref] [PubMed]
- Hickish T, Andre T, Wyrwicz L, et al. MABp1 as a novel antibody treatment for advanced colorectal cancer: a randomised, double-blind, placebo-controlled, phase 3 study. Lancet Oncol 2017;18:192-201. [Crossref] [PubMed]
- Trikha M, Corringham R, Klein B, et al. Targeted anti-interleukin-6 monoclonal antibody therapy for cancer: a review of the rationale and clinical evidence. Clin Cancer Res 2003;9:4653-65. [PubMed]
- Clarke SJ, Smith JT, Gebbie C, et al. A phase I, pharmacokinetic (PK), and preliminary efficacy assessment of ALD518, a humanized anti-IL-6 antibody, in patients with advanced cancer. J Clin Oncol 2009;27:3025.
- Rigas JR, Schuster M, Orlov SV, et al. Efect of ALD518, a humanized anti-IL-6 antibody, on lean body mass loss and symptoms in patients with advanced non-small cell lung cancer (NSCLC): Results of a phase II randomized, double-blind safety and efficacy trial. J Clin Oncol 2010;28:7622. [Crossref]
- Schuster M, Rigas JR, Orlov SV, et al. ALD518, a humanized anti-IL-6 antibody, treats anemia in patients with advanced non-small cell lung cancer (NSCLC): Results of a phase II, randomized, double-blind, placebo-controlled trial. J Clin Oncol 2010;28:7631. [Crossref]
- Bayliss TJ, Smith JT, Schuster M, et al. A humanized anti-IL-6 antibody (ALD518) in non-small cell lung cancer. Expert Opinion on Biological Therapy 2011;11:1663-8. [Crossref] [PubMed]
- Ma JD, Heavey SF, Revta C, et al. Novel investigational biologics for the treatment of cancer cachexia. Expert Opin Biol Ther 2014;14:1113-20. [Crossref] [PubMed]
- Chasen M, Hirschman SZ, Bhargava R. Phase II study of the novel peptide-nucleic acid OHR118 in the management of cancer-related anorexia/cachexia. J Am Med Dir Assoc 2011;12:62-7. [Crossref] [PubMed]
- Ebner N, Werner CG, Doehner W, et al. Recent developments in the treatment of cachexia: highlights from the 6th Cachexia Conference. J Cachexia Sarcopenia Muscle 2012;3:45-50. [Crossref] [PubMed]
- Abstracts of the 6th cachexia conference, Milan, Italy, December 8–10, 2011. J Cachexia Sarcopenia Muscle 2011;2:209-61. [Crossref] [PubMed]
- Abstracts of the 7th Cachexia Conference, Kobe/Osaka, Japan, December 9–11, 2013. J Cachexia Sarcopenia Muscle 2013;4:295-343. [Crossref] [PubMed]