Efficacy of preoxygenation with end-tidal oxygen when using different oxygen concentrations in patients undergoing general surgery: a single-center retrospective observational study
Introduction
Preoxygenation is a simple, but highly important procedure that can increase the body’s oxygen reserve, to prevent arterial desaturation during apnea in the anesthesia induction period. To store oxygen efficiently in the body, tidal volume breathing with 100% oxygen for 3 min is considered the standard technique for preoxygenation (1). A high fraction of inspired oxygen (FiO2) should be delivered to patients without leakage (2). Given that end-tidal oxygen (EtO2) reflects the alveolar fraction of oxygen, EtO2 can be used as an index of adequate preoxygenation, and as a means of monitoring leakage. Adequate preoxygenation is considered to have been achieved when the difference between the FiO2 and EtO2 fractions approaches 10% (2-5).
A higher FiO2 or EtO2 will lead to longer duration of apnea without desaturation (DAWD); however, it may also increase resorption atelectasis (2). Since nitrogen in air is not taken up, the alveoli are kept open by nitrogen. When the nitrogen used to splint alveoli washed out, oxygen absorption exceeds carbon dioxide (CO2) excretion, potentially accelerating resorption atelectasis; this can reduce the functional residual capacity (6), impair gas exchange, and cause respiratory dysfunction and lung injury (7). This may explain why preoxygenation using 100% oxygen precipitates more atelectasis; this would be consistent with Reber et al., who reported that preoxygenation and hyperoxygenation produced more atelectasis (8). To prevent atelectasis, the use of lower FiO2 (9-11) or techniques (12) such as the alveolar recruitment maneuver (ARM) and positive end-expiratory pressure (PEEP) have been suggested (6,13).
However, because a high fraction of oxygen is essential to avoid hypoxia, questions have been raised regarding the optimal oxygen concentration (14-16). While ≤80% oxygen could reduce atelectasis (6,9,14), 80% oxygen does not worsen pulmonary gas exchange, or the lung volumes (17). Despite a modest shortening of DAWD (18), we thought that 80% oxygen could be an alternative to 100% oxygen, if it can enhance the efficacy of preoxygenation. Using 80% oxygen may provide many of the benefits of 100% oxygen (17), while atelectasis is much less than 100% oxygen, but comparable to 30% oxygen (9,17).
Many studies have reported on the time required for EtO2 to reach 90% when using 100% oxygen for preoxygenation (3,19-21); however, few studies have measured the time required for EtO2 to reach 70% when using 80% oxygen. For this reason, we attempted to determine if there is a difference in preoxygenation time, using EtO2 as an endpoint, between different oxygen concentrations (100% vs. 80%). DAWD is naturally shortened when 80% oxygen is used; thus, the occurrence of desaturation was also investigated. We also wanted to determine the proportion of patients for whom >3 min was required to achieve adequate preoxygenation. We present the following article in accordance with the STROBE reporting checklist (available at https://apm.amegroups.com/article/view/10.21037/apm-22-647/rc).
Methods
This was a single-center, retrospective, observational study conducted at a tertiary hospital in Korea. The study was conducted in accordance with the Declaration of Helsinki (as revised in 2013), and was approved by Institutional Review Board (IRB) of Soonchunhyang University Bucheon Hospital (No. 2021-11-033). The requirement for informed consent was waived due to the retrospective nature of the study and anonymity of the data. Patient-identifying data were not collected. The reporting of this study conforms to STROBE guidelines.
Data selection
All data in this study were obtained from medical records and the VitalDB database at our tertiary hospital. Construction of the database was approved by our IRB (IRB No. 2018-06-012) (22). The VitalDB program collects data from multiple anesthesia devices, including patient monitors, anesthesia machines, ventilators, bispectral index (BIS), and target-controlled infusion (TCI) (23). All data collected using multiple monitors are automatically recorded using the Vital Recorder tool; time-synchronized data are shown (24) in Figure S1.
From the VitalDB database, FiO2, EtO2, and capnography data were extracted. The start and end times of preoxygenation, start of anesthesia, and start and end times of intubation were also obtained. Data other than VitalDB data were obtained from anesthesia records; demographic data included age, sex, weight, height, body mass index (BMI), American Society of Anesthesiologists (ASA) physical status, smoking status, concomitant pulmonary diseases such as chronic obstructive pulmonary disease (COPD) and asthma, Cormack-Lehane grade (25) during intubation (grade 1, most of the glottis is visible; grade 2, only the posterior extremity of the glottis is visible; grade 3, no part of the glottis can be seen, but the epiglottis is visible; grade 4, neither the glottis or epiglottis can be seen), and type, site and emergency status of the operation.
Data on patients aged 17–85 years under the care of the Department of General Surgery between February 1, 2021 and November 12, 2021 were reviewed. Bhatia et al. reported that the time taken for EtO2 to reach 90% when using 100% oxygen in young adults was 157±32 s (19). Assuming that a 5% difference in preoxygenation time is clinically significant, 326 patients were estimated to be needed to achieve 80% power with a two-sided significance level of 0.05 using the two-sample t-test. Of the patients who underwent general surgery under general anesthesia, only those with satisfactory EtO2 and capnography data were included. The patients were classified into 100% and 80% groups according to the oxygen concentration used during preoxygenation. From the start to the end of preoxygenation, if a continuous exponential increase in EtO2 was identified, and a capnography waveform of the patient’s respiration was recorded simultaneously (Figure 1), the data were considered sufficient for analysis. Patients whose data were incomplete were excluded, including those with no or irregular EtO2 and capnography data; an absent or irregular EtO2 graph and EtCO2 waveform before anesthesia induction (Figure S2), or no FiO2 or EtO2 data due to technical errors (Figure S3).
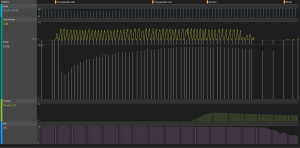
Preoxygenation and induction periods
All preoxygenation and anesthesia methods were performed in accordance with the protocols of our institution. We routinely used 100% oxygen during preoxygenation in the supine position. A head-up or ramped position could be used at the anesthesiologist’s discretion. A tight-fitting face mask without leakage, and the use of 100% oxygen, is essential during preoxygenation, but many anesthesiologists in our institution do not perform preoxygenation properly; sometimes there is a gap between the patients’ face and the face mask, resulting in leakage. This leakage in turn results in the entrainment of >20% room air, thus lowering the FiO2 and EtO2 (26,27). After a journal club session on airway management aimed at reducing atelectasis, we were reminded of the importance of using an appropriately fitting face mask without leakage, using 80% or 100% oxygen at the anesthesiologist’s discretion, with a fresh gas flow rate of 6–10 L/min. We also used EtO2 as an endpoint, rather than the preoxygenation time. This enabled us to collect data using different oxygen concentrations during preoxygenation.
The preoxygenation time was defined as the time between the start and end of preoxygenation. The start of preoxygenation was set as the onset of the capnography waveform (Figure 2). The end of preoxygenation was defined as the time when a 10% difference between FiO2 and EtO2 occurred (EtO2 =90% and 70% for the 100% and 80% groups, respectively); a 10% difference corresponds to an alveolar nitrogen concentration of approximately 5% (4,28) and alveolar CO2 proportion of 5% (4). This is considered to reflect adequate preoxygenation (5). Inadequate preoxygenation was defined as failure to achieve a 10% difference between FiO2 and EtO2 after a sufficient recording time, which suggests leakage.
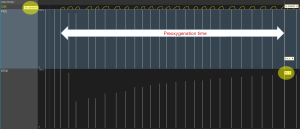
The intubation time was defined as that from the end of the last capnography waveform during face mask ventilation to the start of the first capnography waveform after intubation (Figure 3). We also checked whether there was a desaturation event, defined as a peripheral oxygen saturation (SpO2) lower than 95% or 90% [reflecting “abnormal” and “significant” arterial oxygen desaturation, respectively (29-31)]. The time from the start of propofol administration to the end of intubation was defined as the total induction time.
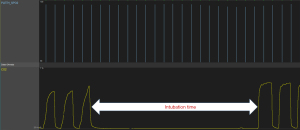
Outcomes
The primary outcome was comparing the preoxygenation time between the 100% and 80% groups to evaluate the efficacy of preoxygenation. Secondary outcomes were the occurrence of a desaturation event during induction period, the ratio of inadequate preoxygenation, and a time more than 3 min to achieve adequate preoxygenation. The Cormack-Lehane grades were also compared between the two groups.
Statistical analysis
All continuous variables were assessed for the normality of their distribution using the Shapiro-Wilk test. Normal continuous variables were analyzed using the independent t-test and are expressed as the means ± standard deviation (SD). Non-normal continuous variables were analyzed using the Mann-Whitney U test and are expressed as median [interquartile range (IQR)]. Categorical variables were analyzed using the chi-square test or Fisher’s exact test as appropriate, and are expressed as numbers (percentages). Propensity score matching (PSM) was performed thorough a logistic regression analysis to reduce selection biasand control of potential confounding factors including age, sex, BMI, ASA physical status, smoking status, COPD, asthma, basal SpO2, abdominal surgery, elective surgery, and the Cormack-Lehane grade. After PSM, continuous variables were analyzed using Wilcoxon signed-rank test and categorical variables were analyzed using the McNemar test or McNemar-Bowker test, as appropriate. Binary logistic regression was used to identify risk factors for inadequate preoxygenation, and a difficult airway. The independent variables were age, sex, BMI, and ASA physical status. A P value <0.05 was considered to indicate statistical significance. Statistical analyzes were performed using IBM SPSS Statistics (ver. 26.0; IBM, Armonk, NY, USA).
Results
The study reviewed 1,377 patients under the care of the Department of General Surgery between February 1, 2021 and November 12, 2021. After excluding 1,047 patients due to insufficient data (891 patients, no or an irregular EtO2 graph and capnography waveform; 156 patients, missing data due to technical errors), data from 330 patients were extracted to analyze the efficacy of preoxygenation (Figure 4). Of these, 179 patients were classified into the 80% group and 151 into the 100% group, according to the oxygen concentration. After PSM, 143 patients in each group (total of 286 patients) were matched.
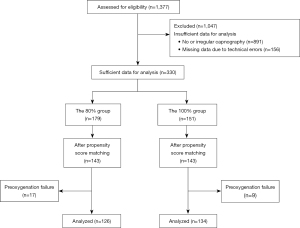
Demographic data before and after PSM are presented in Table 1. Before PSM, there were no significant differences in age, sex, weight, height, BMI, the proportion of smokers, COPD, asthma, abdominal surgery and emergency surgery, or basal SpO2 between the two groups. However, there was a significant difference in ASA physical status (P<0.05). After PSM, none of the demographic characteristics differed between the two groups.
Table 1
Variables | Before PSM | After PSM | |||||||
---|---|---|---|---|---|---|---|---|---|
Total (n=330) | 80% group (n=179) | 100% group (n=151) | P value | Total (n=286) | 80% group (n=143) | 100% group (n=143) | P value | ||
Sex (male:female) | 143:187 (43%:57%) | 78:101 (44%:56%) | 65:86 (43%:57%) | 0.923† | 122:164 (43%:57%) | 62:81 (43%:57%) | 60:83 (42%:58%) | 0.899¶ | |
Age (years) | 58.5 [48, 66] | 59 [51, 69] | 58.5 [47, 65] | 0.174‡ | 58 [48, 67.5] | 57.5 [48.8, 68] | 58.5 [44.5, 65] | 0.626¶ | |
Weight (kg) | 63.0 [56.1, 72.1] | 63.0 [54.7, 70.8] | 63.4 [57.3, 72.9] | 0.105‡ | 62.7 [55.9, 71.7] | 62.3 [54.7, 70.1] | 64.0 [57.3, 73.6] | 0.084¶ | |
Height (cm) | 160.5 [154.5, 167.0] | 160.0 [153.9, 165.4] | 160.6 [155.4, 168.6] | 0.138‡ | 160.4 [154.5, 166.9] | 160.1 [153.2, 165.5] | 160.6 [155.4, 168.4] | 0.175†† | |
BMI (kg/m2) | 24.7 [22.1, 26.7] | 24.9 [22.1, 26.9] | 24.4 [22.4, 26.6] | 0.879‡ | 24.5 [21.8, 26.7] | 24.7 [21.6, 26.6] | 24.7 [22.4, 26.9] | 0.459†† | |
ASA classification (I:II:III:IV) | 97:161:71:1 (29%:49%:22%:0%) | 41:95:42:1 (23%:53%:23%:1%) | 56:66:29:0 (37%:44%:19%:0%) | 0.035§ | 91:136:59:0 (32%:48%:21%:0%) | 39:73:31:0 (27%:51%:22%:0%) | 52:63:28:0 (36%:44%:20%:0%) | 0.122‡‡ | |
Smoking (none:current:ex) | 277:35:18 (84%:11%:5%) | 153:18:8 (85%:10%:4%) | 124:17:10 (82%:11%:7%) | 0.632† | 240:30:16 (84%:10%:6%) | 121:14:8 (85%:10%:6%) | 119:16:8 (83%:11%:6%) | 0.860‡‡ | |
COPD | 8 (2%) | 3 (2%) | 5 (3%) | 0.477§ | 6 (2%) | 3 (2%) | 3 (2%) | 1.000¶ | |
Asthma | 17 (5%) | 12 (7%) | 5 (3%) | 0.165† | 11 (4%) | 6 (4%) | 5 (3%) | 1.000¶ | |
Basal SpO2 | 97 [97, 98] | 97 [96, 98] | 97 [97, 98] | 0.240‡ | 97 [97, 98] | 97.5 [99, 98] | 97 [97, 98] | 0.396†† | |
Abdominal surgery | 278 (84%) | 151 (84%) | 127 (84%) | 0.950† | 240 (84%) | 120 (84%) | 120 (84%) | 1.000¶ | |
Emergency surgery | 5 (2%) | 2 (1%) | 3 (2%) | 0.664‡ | 4 (1%) | 2 (1%) | 2 (1%) | 1.000¶ |
The values are median [1Q, 3Q] or number (%). †, Chi-square test; ‡, Mann-Whitney U test; §, Fisher’s exact test; ¶, McNemar test; ††, Wilcoxon signed-rank test; ‡‡, McNemar-Bowker test. PSM, propensity score matching; BMI, body mass index; ASA, American Society of Anesthesiologists; COPD, chronic obstructive pulmonary disease; SpO2, peripheral oxygen saturation.
After PSM, the preoxygenation time also did not differ significantly between the two groups [median difference =−1; 95% confidence interval (CI): −13 to 10; P=0.605]. There were also no differences in the overall rate of inadequate preoxygenation (12% vs. 6%, P=0.143) or the proportion of patients for whom >3 min was required for adequate preoxygenation (26% vs. 28%, P=0.118) (Table 2). No demographic characteristics were associated with inadequate preoxygenation.
Table 2
Variables | After PSM | |||||
---|---|---|---|---|---|---|
Total (n=286) | 80% group (n=143) | 100% group (n=143) | P value | Median difference | 95% CI | |
Preoxygenation time (s) | – | 143 [120.5, 181.5] | 144 [109.75, 186.25] | 0.605† | −1 | −13 to 10 |
Preoxygenation success/failure | 260:26 (91%:9%) | 126:17 (88%:12%) | 134:9 (94%:6%) | 0.143‡ | – | – |
Time for adequate preoxygenation (≤3 min:>3 min) | 190:70 (73%:27%) | 93:33 (74%:26%) | 97:37 (72%:28%) | 0.118‡ | – | – |
SpO2 <95% | 3 (1%) | 1 (0.7%) | 2 (1.4%) | 1.000‡ | – | – |
SpO2 <90% | 1 (0.3%) | 0 (0%) | 1 (0.7%) | 0.143‡ | – | – |
Minimal SpO2 | 100 [99, 100] | 100 [99, 100] | 100 [99, 100] | 0.136† | 0 | −5 to 0 |
Intubation time (s) | 41 [35, 49] | 41 [35, 49] | 40 [35, 48] | 0.772† | 0 | −3 to 2 |
Cormack-Lehane grade (1:2:3:4) | 168:91:22:4 (59%:32%:8%:1%) | 96:38:8:1 (67%:27%:6%:1%) | 72:53:14:3 (50%:37%:10%:2%) | 0.004§ | – | – |
Total induction time (s) | 354 [323, 407] | 348 [325, 417] | 365 [321, 418] | 0.071† | −14.5 | −30.5 to 1.5 |
The data are the median [IQR] or number (%). †, Wilcoxon signed-rank test; ‡, McNemar test; §, McNemar-Bowker test. PSM, propensity score matching; CI, confidence interval; SpO2, peripheral oxygen saturation; IQR, interquartile range.
There was only one case of significant arterial oxygen desaturation (SpO2 <90%), of a 39-year-old woman (height, 155 cm; weight, 110 kg; BMI, 45.8 kg/m2; basal SpO2, 95%; current smoker, 10 pack-years) scheduled for a laparoscopic cholecystectomy. She breathed 100% oxygen until the EtO2 reached >90% (preoxygenation time: 64 s). However, her SpO2 fell gradually to 88% after the propofol infusion. Despite facemask ventilation, the SpO2 remained at 90%. Her Cormack-Lehane grade was 4 and tracheal intubation was attempted at least twice, judging from the capnography waveform (Figure S4). The SpO2 fell to 78% during the second intubation attempt, but recovered to 97% after intubation.
Despite the significant group difference in laryngeal grade (P=0.004 after PSM), the intubation time did not differ significantly between the two groups (P=0.772 after PSM). Assuming that Cormack-Lehane grades 1 and 2 represent easy intubation, and grades 3 and 4 difficult intubation (32), the rate of difficult airway was 9.1% (26 of 285 patients) after PSM. BMI [odds ratio (OR) =1.14; 95% CI: 1.04 to 1.24; P=0.004 after PSM] was identified as a risk factor for difficult airway by logistic regression.
Discussion
We found that the preoxygenation time, defined as the time taken to achieve a 10% difference between FIO2 and EtO2, did not differ significantly between the 100% and 80% groups (median difference =−1; 95% CI: −13 to 10 after PSM). To our knowledge, no studies have compared the time taken for adequate preoxygenation using 100% and 80% oxygen, although this result may be a result of the physiological law of exponential wash-in (33). In addition, our endpoint was the time required for a 10% difference between FiO2 and EtO2, not 3 min; other studies have tended to report the EtO2 at 3 min after preoxygenation (4,19,34). Studies have reported the time required for EtO2 to reach 90% when using 100% oxygen (3,35), but we uniquely also measured the time required for EtO2 to reach 70% when using 80% oxygen. Tidal volume breathing for 3 min, considered a standard technique for preoxygenation, did not achieve adequate preoxygenation in 27% of our patients after PSM. Using 90% EtO2 as the endpoint when applying 100% oxygen during preoxygenation, Machlin et al. reported that it took 154 (range, 43–364) s to achieve adequate preoxygenation (3), while Bhatia et al. reported that it took 157 (range, 54–180) s in young adults and 164 (range, 110–180) s in elderly patients (19). These values are similar to those in our study, of 152 (range, 77–356) s and 151 (range, 60–288) s after PSM for the 80% and 100% groups, respectively; however, the data were not normally distributed. In our study, the preoxygenation time was in accordance with the EtO2, which is an objective indicator, rather than the 3 min used is routine in actual clinical practice.
Guidelines for anticipated and unanticipated difficult intubation recommend that all patients should be preoxygenated before airway manipulation (5,36-38). However, few anesthesiologists routinely perform preoxygenation (39); in fact, it is frequently neglected (40). Although the EtO2 is a ventilator parameter used in clinical practice, it is not monitored routinely (41). The importance of EtO2 has been emphasized in emergency departments, wherein rapid sequence intubation is commonly performed (42,43); EtO2 monitoring improved the quality of preoxygenation, resulting in a reduction in hypoxemic events during intubation (44). Adequate preoxygenation confirmed by EtO2 monitoring will improve patient safety by checking for the presence of a leak and optimizing oxygen wash-in, which makes the induction of anesthesia more comfortable and relaxed.
The frequency of inadequate preoxygenation was reported to be 11.5% (23 out of 200 patients) by Machlin et al. (3). In our study, 9% of the patients (26 of 286) had inadequate preoxygenation after PSM. Leakage is suspected when there is either no capnography waveform or the EtO2 is lower than expected. Perhaps the rate was lower in our study because we checked only for “a failure to reach a 10% difference between FiO2 and EtO2” among patients in whom complete capnography was acquired.
Machlin et al. reported that, for 26% (46 of 177) of their patients, >3 min was required for adequate preoxygenation (3), while Berry et al. reported that this applied to 22.5% (9 of 40) of patients (4). For 27% (70 out of 260) of our patients it took >180 s after PSM to achieve adequate preoxygenation. Thus, 3-min preoxygenation is not sufficient for some patients. EtO2 can be a useful indicator of whether adequate preoxygenation has been attained or whether leakage is present (41,43).
As the technique has improved, more comfortable and reliable equipment has been commercialized to improve patient safety, including a videolaryngoscope. We routinely used a videolaryngoscope during intubation, which improves intubation time in difficult airways compared with direct laryngoscopy (45-47). This may explain why there was no significant group difference in intubation time in our study, despite a significant difference in Cormack-Lehane grade. However, since the risk of difficult mask ventilation and intubation is unpredictable and we never know when we will encounter such situations, it is important to administer oxygen accurately, monitor how much oxygen is delivered, and begin the induction of anesthesia. EtO2 monitoring might be an essential element of preoxygenation and is of sufficient value for continuous monitoring and evaluation.
Since nitrogen in air is not taken up, the alveoli are kept open by nitrogen. This may explain why preoxygenation using 100% oxygen precipitates more atelectasis; this would be consistent with Reber et al., who reported that preoxygenation and hyperoxygenation produced more atelectasis (8). However, because a high fraction of oxygen is essential to avoid hypoxia, questions have been raised regarding the optimal oxygen concentration (14-16). While ≤80% oxygen could reduce atelectasis (6,9,14), 80% oxygen does not worsen pulmonary gas exchange, or the lung volumes (17). Despite a modest shortening of DAWD (18), we thought that 80% oxygen could be an alternative to 100% oxygen, if it can enhance the efficacy of preoxygenation. However, there was no difference in the adequate preoxygenation rate between 80% and 100% oxygen, which may be a result of the physiological law of exponential wash-in.
Although there was only one desaturation case in our series, our sample was small, and difficult mask ventilation or intubation cannot be predicted. Many studies reduced atelectasis using 100% oxygen by applying continuous positive airway pressure (CPAP), the ARM, or PEEP (12,48-51). In addition, the median total induction time was 353.5 s (IQR: 322.75–406.5 s) after PSM. Edmark et al. reported DAWD values of 414±84 and 303±59 s using 100% and 80% oxygen, respectively (9). Based on those values, only 75% and <50% of the patients would be able to endure in the event of “cannot intubate, cannot oxygenate” when using 100%, and 80% oxygen, respectively. Therefore, 100% oxygen should be used during preoxygenation with CPAP, ARM and PEEP to reduce atelectasis, until a large study proves that 80% oxygen differs in safety from 100% oxygen during the induction period.
There are several limitations to our study. First, our study was retrospective, and the method of preoxygenation was not protocolized, and was adjusted at the anesthesiologist’s discretion. And the period of data collection differed, so selection bias is inevitable. The choice of which oxygen concentration to be used could also affect. For those reasons, we performed PSM to reduce selection bias, but many patients were already excluded because we analyzed only those with objective data, and there was still a significant difference in Cormak-Lehane grade between the two groups. Second, there may also have been technical problems in performing preoxygenation, although they might have been insignificant because we selected only patients with sufficient data for analysis. The preoxygenation time was influenced by several factors, such as position (supine, head-up or ramped) or respiratory pattern (minute ventilation). Some patients breathed shallowly and rapidly, while others breathed deeply and slowly; hyperventilation can achieve adequate preoxygenation in a short period of time (52). Precise tidal volume breathing, through education or guidance of patients, would improve preoxygenation times. Further protocolized and randomized studies were needed to confirm our results. Third, we could not measure DAWD due to the retrospective nature of the study; DAWD measurements are not performed routinely in actual clinical practice. However, the efficiency of preoxygenation is also important when evaluating the effectiveness of preoxygenation. We are currently conducting a prospective study to evaluate the effectiveness of preoxygenation. Finally, the independent variables in the logistic regression analysis were written demographic characteristics. Baillard et al. reported that the main predictors (OR >9) of inadequate preoxygenation are the FiO2, an ASA score of 4, and facial hair (53). In our study, these risk factors may not have been present due to the small sample size and limited number of variables. More attention should be paid to patients with these factors, as they may overlap with the risk factors for difficult mask ventilation (44,53).
Conclusions
In conclusion, we found no difference in preoxygenation time between 100% and 80% oxygen, using EtO2 as an end-point. Currently, preoxygenation for 3 min is considered the standard, but many patients required more than 3 min for adequate preoxygenation. Therefore, it is helpful to use EtO2 to confirm adequate preoxygenation. EtO2 monitoring would complement evaluations of preoxygenation adequacy.
Acknowledgments
This manuscript is a revision of the first author’s master’s thesis. This manuscript is the authors’ original work and has not been previously published elsewhere.
Funding: This study was supported by the Soonchunhyang University Research Fund.
Footnote
Reporting Checklist: The authors have completed the STROBE reporting checklist. Available at https://apm.amegroups.com/article/view/10.21037/apm-22-647/rc
Peer Review File: Available at https://apm.amegroups.com/article/view/10.21037/apm-22-647/prf
Conflicts of Interest: All authors have completed the ICMJE uniform disclosure form (available at https://apm.amegroups.com/article/view/10.21037/apm-22-647/coif). The authors have no conflicts of interest to declare.
Ethical Statement: The authors are accountable for all aspects of the work in ensuring that questions related to the accuracy or integrity of any part of the work are appropriately investigated and resolved. The study was conducted in accordance with the Declaration of Helsinki (as revised in 2013). The study was approved by IRB of Soonchunhyang University Buchoen Hospital (IRB No. 2021-11-033) and individual consent for this retrospective analysis was waived due to the retrospective nature of the study and anonymity of the data.
Open Access Statement: This is an Open Access article distributed in accordance with the Creative Commons Attribution-NonCommercial-NoDerivs 4.0 International License (CC BY-NC-ND 4.0), which permits the non-commercial replication and distribution of the article with the strict proviso that no changes or edits are made and the original work is properly cited (including links to both the formal publication through the relevant DOI and the license). See: https://creativecommons.org/licenses/by-nc-nd/4.0/.
References
- Bouroche G, Bourgain JL. Preoxygenation and general anesthesia: a review. Minerva Anestesiol 2015;81:910-20. [PubMed]
- Nimmagadda U, Salem MR, Crystal GJ. Preoxygenation: Physiologic Basis, Benefits, and Potential Risks. Anesth Analg 2017;124:507-17. [Crossref] [PubMed]
- Machlin HA, Myles PS, Berry CB, et al. End-tidal oxygen measurement compared with patient factor assessment for determining preoxygenation time. Anaesth Intensive Care 1993;21:409-13. [Crossref] [PubMed]
- Berry CB, Myles PS. Preoxygenation in healthy volunteers: a graph of oxygen "washin" using end-tidal oxygraphy. Br J Anaesth 1994;72:116-8. [Crossref] [PubMed]
- Kornas RL, Owyang CG, Sakles JC, et al. Evaluation and Management of the Physiologically Difficult Airway: Consensus Recommendations From Society for Airway Management. Anesth Analg 2021;132:395-405. [Crossref] [PubMed]
- Hedenstierna G, Edmark L. Mechanisms of atelectasis in the perioperative period. Best Pract Res Clin Anaesthesiol 2010;24:157-69. [Crossref] [PubMed]
- Lagier D, Zeng C, Fernandez-Bustamante A, et al. Perioperative Pulmonary Atelectasis: Part II. Clinical Implications. Anesthesiology 2022;136:206-36. [Crossref] [PubMed]
- Reber A, Engberg G, Wegenius G, et al. Lung aeration. The effect of pre-oxygenation and hyperoxygenation during total intravenous anaesthesia. Anaesthesia 1996;51:733-7. [PubMed]
- Edmark L, Kostova-Aherdan K, Enlund M, et al. Optimal oxygen concentration during induction of general anesthesia. Anesthesiology 2003;98:28-33. [Crossref] [PubMed]
- Kim BR, Lee S, Bae H, et al. Lung ultrasound score to determine the effect of fraction inspired oxygen during alveolar recruitment on absorption atelectasis in laparoscopic surgery: a randomized controlled trial. BMC Anesthesiol 2020;20:173. [Crossref] [PubMed]
- Kim HI, Min JY, Lee JR, et al. The effect of oxygen concentration on atelectasis formation during induction of general anesthesia in children: A prospective randomized controlled trial. Paediatr Anaesth 2021;31:1276-81. [Crossref] [PubMed]
- Rusca M, Proietti S, Schnyder P, et al. Prevention of atelectasis formation during induction of general anesthesia. Anesth Analg 2003;97:1835-9. [Crossref] [PubMed]
- Neumann P, Rothen HU, Berglund JE, et al. Positive end-expiratory pressure prevents atelectasis during general anaesthesia even in the presence of a high inspired oxygen concentration. Acta Anaesthesiol Scand 1999;43:295-301. [Crossref] [PubMed]
- O'Brien J. Absorption atelectasis: incidence and clinical implications. AANA J 2013;81:205-8. [PubMed]
- Hedenstierna G. Airway closure, atelectasis and gas exchange during anaesthesia. Minerva Anestesiol 2002;68:332-6. [PubMed]
- Edmark L, Auner U, Enlund M, et al. Oxygen concentration and characteristics of progressive atelectasis formation during anaesthesia. Acta Anaesthesiol Scand 2011;55:75-81. [Crossref] [PubMed]
- Akça O, Podolsky A, Eisenhuber E, et al. Comparable postoperative pulmonary atelectasis in patients given 30% or 80% oxygen during and 2 hours after colon resection. Anesthesiology 1999;91:991-8. [Crossref] [PubMed]
- Hans GA, Sottiaux TM, Lamy ML, et al. Ventilatory management during routine general anaesthesia. Eur J Anaesthesiol 2009;26:1-8. [Crossref] [PubMed]
- Bhatia PK, Bhandari SC, Tulsiani KL, et al. End-tidal oxygraphy and safe duration of apnoea in young adults and elderly patients. Anaesthesia 1997;52:175-8. [Crossref] [PubMed]
- Mathew G, Manjuladevi M, Joachim N, et al. Effect of high fresh gas flow and pattern of breathing on rapid preoxygenation. Indian J Anaesth 2022;66:213-9. [Crossref] [PubMed]
- Arora S, Gupta P, Arya VK, et al. Efficacy of preoxygenation using tidal volume breathing: a comparison of Mapleson A, Bain's and Circle system. Braz J Anesthesiol 2018;68:128-34. [Crossref] [PubMed]
- Lee J, Woo J, Kang AR, et al. Comparative Analysis on Machine Learning and Deep Learning to Predict Post-Induction Hypotension. Sensors (Basel) 2020;20:4575. [Crossref] [PubMed]
- Lee HC, Ryu HG, Chung EJ, et al. Prediction of Bispectral Index during Target-controlled Infusion of Propofol and Remifentanil: A Deep Learning Approach. Anesthesiology 2018;128:492-501. [Crossref] [PubMed]
- Lee HC, Jung CW. Vital Recorder-a free research tool for automatic recording of high-resolution time-synchronised physiological data from multiple anaesthesia devices. Sci Rep 2018;8:1527. [Crossref] [PubMed]
- Cormack RS, Lehane J. Difficult tracheal intubation in obstetrics. Anaesthesia 1984;39:1105-11. [Crossref] [PubMed]
- McGowan P, Skinner A. Preoxygenation--the importance of a good face mask seal. Br J Anaesth 1995;75:777-8. [Crossref] [PubMed]
- Gagnon C, Fortier LP, Donati F. When a leak is unavoidable, preoxygenation is equally ineffective with vital capacity or tidal volume breathing. Can J Anaesth 2006;53:86-91. [Crossref] [PubMed]
- Hamilton WK, Eastwood DW. A study of denitrogenation with some inhalation anesthetic systems. Anesthesiology 1955;16:861-7. [Crossref] [PubMed]
- Davis DP, Lemieux J, Serra J, et al. Preoxygenation reduces desaturation events and improves intubation success. Air Med J 2015;34:82-5. [Crossref] [PubMed]
- Considine J. The reliability of clinical indicators of oxygenation: a literature review. Contemp Nurse 2005;18:258-67. [Crossref] [PubMed]
- O'Driscoll BR, Howard LS, Earis J, et al. BTS guideline for oxygen use in adults in healthcare and emergency settings. Thorax 2017;72:ii1-90. [Crossref] [PubMed]
- Shiga T, Wajima Z, Inoue T, et al. Predicting difficult intubation in apparently normal patients: a meta-analysis of bedside screening test performance. Anesthesiology 2005;103:429-37. [Crossref] [PubMed]
- Nimmagadda U, Salem MR, Joseph NJ, et al. Efficacy of preoxygenation with tidal volume breathing. Comparison of breathing systems. Anesthesiology 2000;93:693-8. [Crossref] [PubMed]
- Kang H, Park HJ, Baek SK, et al. Effects of preoxygenation with the three minutes tidal volume breathing technique in the elderly. Korean J Anesthesiol 2010;58:369-73. [Crossref] [PubMed]
- Nimmagadda U, Chiravuri SD, Salem MR, et al. Preoxygenation with tidal volume and deep breathing techniques: the impact of duration of breathing and fresh gas flow. Anesth Analg 2001;92:1337-41. [Crossref] [PubMed]
- Henderson JJ, Popat MT, Latto IP, et al. Difficult Airway Society guidelines for management of the unanticipated difficult intubation. Anaesthesia 2004;59:675-94. [Crossref] [PubMed]
- Frerk C, Mitchell VS, McNarry AF, et al. Difficult Airway Society 2015 guidelines for management of unanticipated difficult intubation in adults. Br J Anaesth 2015;115:827-48. [Crossref] [PubMed]
- Apfelbaum JL, Hagberg CA, Connis RT, et al. 2022 American Society of Anesthesiologists Practice Guidelines for Management of the Difficult Airway. Anesthesiology 2022;136:31-81. [Crossref] [PubMed]
- Heck Z, Stegmann JU, Lorenz C, et al. Acceptance of preoxygenation in clinical routine by patients and by anaesthesiologists. Anasthesiol Intensivmed Notfallmed Schmerzther 2001;36:471-5. [Crossref] [PubMed]
- Chen ST, Min S. Oxygen reserve index, a new method of monitoring oxygenation status: what do we need to know? Chin Med J (Engl) 2020;133:229-34. [Crossref] [PubMed]
- Slinger PD. Is There Anything New About Preoxygenation? Duh, Yeah! Anesth Analg 2017;124:388-9. [Crossref] [PubMed]
- Oliver M, Caputo ND, West JR, et al. Emergency physician use of end-tidal oxygen monitoring for rapidsequence intubation. J Am Coll Emerg Physicians Open 2020;1:706-13. [Crossref] [PubMed]
- Jarvis JL, King T. Is adequate preoxygenation about more than just 3 minutes? J Am Coll Emerg Physicians Open 2020;1:714-5. [Crossref] [PubMed]
- Baillard C, Boubaya M, Statescu E, et al. Incidence and risk factors of hypoxaemia after preoxygenation at induction of anaesthesia. Br J Anaesth 2019;122:388-94. [Crossref] [PubMed]
- Savoldelli GL, Schiffer E, Abegg C, et al. Comparison of the Glidescope, the McGrath, the Airtraq and the Macintosh laryngoscopes in simulated difficult airways*. Anaesthesia 2008;63:1358-64. [Crossref] [PubMed]
- Malik MA, O'Donoghue C, Carney J, et al. Comparison of the Glidescope, the Pentax AWS, and the Truview EVO2 with the Macintosh laryngoscope in experienced anaesthetists: a manikin study. Br J Anaesth 2009;102:128-34. [Crossref] [PubMed]
- Lim Y, Yeo SW. A comparison of the GlideScope with the Macintosh laryngoscope for tracheal intubation in patients with simulated difficult airway. Anaesth Intensive Care 2005;33:243-7. [Crossref] [PubMed]
- Östberg E, Auner U, Enlund M, et al. Minimizing atelectasis formation during general anaesthesia-oxygen washout is a non-essential supplement to PEEP. Ups J Med Sci 2017;122:92-8. [Crossref] [PubMed]
- Généreux V, Chassé M, Girard F, et al. Effects of positive end-expiratory pressure/recruitment manoeuvres compared with zero end-expiratory pressure on atelectasis during open gynaecological surgery as assessed by ultrasonography: a randomised controlled trial. Br J Anaesth 2020;124:101-9. [Crossref] [PubMed]
- Delay JM, Sebbane M, Jung B, et al. The effectiveness of noninvasive positive pressure ventilation to enhance preoxygenation in morbidly obese patients: a randomized controlled study. Anesth Analg 2008;107:1707-13. [Crossref] [PubMed]
- Coussa M, Proietti S, Schnyder P, et al. Prevention of atelectasis formation during the induction of general anesthesia in morbidly obese patients. Anesth Analg 2004;98:1491-5. table of contents. [Crossref] [PubMed]
- Benumof JL. Preoxygenation: best method for both efficacy and efficiency. Anesthesiology 1999;91:603-5. [Crossref] [PubMed]
- Baillard C, Depret F, Levy V, et al. Incidence and prediction of inadequate preoxygenation before induction of anaesthesia. Ann Fr Anesth Reanim 2014;33:e55-8. [Crossref] [PubMed]