Blosozumab in the treatment of postmenopausal women with osteoporosis: a systematic review and meta-analysis
Introduction
Osteoporosis is a common chronic senile disease which has a high incidence and mortality rate and a considerable impact on our society. Nearly 200 million people worldwide suffer from osteoporosis. Among them, there are 83.9 million osteoporosis patients in China, which is expected to increase to 212 million by the middle of this century (1). Osteoporosis is a systemic disease characterized by low bone mass and bone structure deterioration, which can increase bone fragility and fracture sensitivity. The World Health Organization defines osteoporosis as bone mineral density (BMD) T-score of less than −2.5 and bone mass reduction between −1.0 and −2.5 (2).
Osteoporosis is caused by several factors, including menopause, aging, genetics, glucocorticoid and aromatase inhibitor use, and long-term bone disuse. The most common cause of osteoporosis in postmenopausal women is hormone imbalance, which leads to high conversion loss and insufficient bone formation. Epidemiologically, osteoporotic fractures occur in 20% of men and 30% of women aged over 50 years. With aging (3), the organs in the body are in a state of degeneration and physical activity is decreased, which lead to an imbalance in the internal environment of the skeleton, namely, an increase in bone destruction and a decrease in bone formation (4). Measurement of BMD is an important method in the diagnosis of osteoporosis. In some patients with brittle fractures, the T-score of BMD is higher than the diagnostic range of osteoporosis (5).
The treatment strategies for osteoporosis include life-style management and medication management (6). Currently, drugs used to manage osteoporosis include bisphosphonates (7), receptor activator of nuclear factor kappa-Β ligand (RANKL) inhibitors (8), selective estrogen receptor modulators (9), teriparatide (10), and abaloparatide (11). Romosozumab and blosozumab are also potential treatment options (12). Blosozumab is a humanized monoclonal antibody against immunoglobulin G4 (IgG4) that binds directly to sclerostin (13), which is an inhibitor of the Wnt signaling pathway (14). The Wnt signaling pathway is involved in several diseases, including bone diseases (15).
The therapeutic potential of antibodies directed against sclerostin has been supported by animal studies. Ovariectomized rats treated with sclerostin antibody for 5 weeks showed a considerable increase in bone formation with reversal of bone loss (16). A further study showed increased bone density at fracture sites in mice treated with a sclerostin inhibitor compared with that in controls (17). Recently, phase I and II clinical trials of blosozumab have been completed (18), and the efficacy is not categorical.
Although preclinical and clinical trials have demonstrated blosozumab’s therapeutic effects in increasing BMD, there has not been a systematic meta-analysis of blosozumab’s role in bone mass enhancement in postmenopausal women. We present the following article in accordance with the PRISMA reporting checklist (19) (available at https://apm.amegroups.com/article/view/10.21037/apm-22-998/rc).
Methods
Protocol
The design of the meta-analysis has been registered in the PROSPERO registry (CRD42020145839). The study was conducted according to the Cochrane handbook for systematic evaluation of interventions (20).
Literature search
The PubMed, Cochrane Library, ClinicalKey, and Embase databases were searched from the inception date to July 01, 2022. We used the keywords “osteoporosis”, “decreased bone mass”, and “blosozumab” to retrieve studies on the relationship between blosozumab and osteoporosis in each database. A detailed search strategy is shown in Figure 1. There was no restriction on publishing language, and the studies included were randomized controlled trials (RCTs) with no limits on the mode of randomization and blinding.
Inclusion criteria
The inclusion criteria were: (I) RCTs comparing the treatment of osteoporosis with blosozumab and a placebo or without treatment, (II) studies on postmenopausal women aged over 50 years, and (III) studies providing bone mineral density data.
The exclusion criteria were: (I) RCTs without a placebo or without treatment, (II) clinical studies of patients with glucocorticoid-induced secondary osteoporosis; and (III) clinical trials of different combinations of osteoporosis drugs and blosozumab in the treatment of osteoporosis.
Risk of bias assessment
The quality of all RCTs included in this study was assessed by 2 researchers in accordance with the Cochrane risk manual independently (Cochrane RoB 2.0). The Cochrane manual includes sequence generation, allocation concealment, blinding, incomplete outcome data, selective outcome reporting, and other sources of bias. The quality indicators were divided into the following 3 categories after standardization: low, high, and some concerns. The studies were classified into high, some concerns, and low quality according to the following criteria: (I) if randomization of sequence generation and allocation concealment were identified as high risk of bias, we categorized the study as low quality; (II) when randomization and allocation concealment were considered low risk of bias, the study was categorized as high quality; and (III) if neither of the above criteria were met, the study was categorized as some concerns quality.
Data extraction
Two researchers independently extracted data from the studies, including the first author name, date of publication, country, patients’ age, sample size, test method, test site, experimental design, drug dose, and follow-up time. If there were 2 or more groups of data in a selected article, only the data to be analyzed were extracted. Conflicts were resolved through negotiation. If the original data were not provided in the paper, they were obtained from the original polyline graph using GETDATA software. Our primary outcome was lumbar bone marrow density, and the biomarkers procollagen type I N-terminal propeptide (P1NP), C-terminal telopeptide of type I collagen (CTX), bone-specific alkaline phosphatase (BSAP), and osteocalcin (OC).
Statistical analysis
Revman 5.3.5 was used to analyze the extracted data. Mean difference (MD) with 95% confidence interval (CI) was adopted for continuous variables, while risk ratio (RR) with 95% CI was used for binary variables. Data heterogeneity was calculated using Cochrane’s Q and I2 statistic by Revman 5.3.5. When I2 was >50% and P was <0.1, we adopted a random-effects model, and when P was >0.1 and I2 was <50%, we adopted a fixed-effect model (20). Heterogeneity may come from population, test method, race and other factors.
Results
Literature search results
In total, 157 articles were retrieved from the PubMed, Embase, ClinicalKey, and Cochrane Library databases. After removing duplicate articles, the number of articles was 109. After excluding articles without full text, there were 83 articles. Three randomized clinical studies (13,18,21) were selected after screening with the inclusion and exclusion criteria. After reading the full text, those 3 articles were included in the present meta-analysis.
Description of included trials
A total of 105 patients were included in the 3 randomized trials; 2 of these trials reported the same data, and therefore we considered them as one trial. The basic information of the included articles is summarized in Table 1.
Table 1
Study | Journal | Country | Design | Sample size | Relevant outcomes | |
---|---|---|---|---|---|---|
Trial group | Control group | |||||
McColm 2014 (13) | Journal of Bone and Mineral Research | USA | RCT, DB | 30 | 29 | BMD, adverse events, t-score, P1NP, CTX, BSAP, osteocalcin |
Recker 2015 (18) | Journal of Bone and Mineral Research | USA | RCT, DB | 11 | 12 | BMD, adverse events, t-score, P1NP, CTX, BSAP, osteocalcin |
Recknor 2015 (21) | Journal of Bone and Mineral Research | USA | RCT, DB | 11 | 12 | BMD, adverse events, t-score, P1NP, CTX, BSAP, osteocalcin |
RCT, randomized controlled trial; DB, double-blind; BMD, bone mineral density; P1NP, procollagen type 1 amino-terminal propeptide; CTX, C-terminal telopeptide of type I collagen; BSAP, bone-specific alkaline phosphatase, OC, osteocalcin.
Risk of bias of the included studies
Risk assessment information is presented in Figures 2,3.
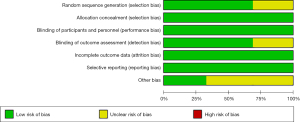
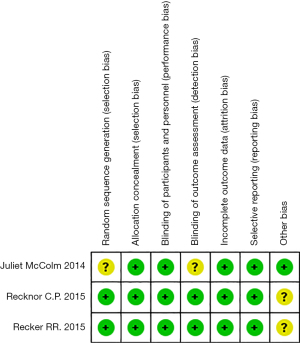
Sequence generation
Two of the 3 randomized trials described how the sequences were generated. McColm [2014] only described the experiment as random and described how it was random. Recker [2015] and Recknor [2015] described that the random numbers were generated using a computer; therefore, their studies were evaluated as low-risk, whereas the McColm study was evaluated as unclear-risk.
Allocation concealment
The 3 randomized trials used the same drugs and same packaging, which did not affect the trials. Therefore, we classified them as low-risk.
Blinding
All 3 trials described a blind approach, in which both the patient and experimenter were blinded, which we considered low risk.
The unreported data of McColm [2014] (13), Recker [2015] (21), and Recknor [2015] (18) were balanced, and the reasons for missing data were similar. Therefore, we considered these RCTs as having a low-risk bias.
Selective reporting
The protocol and results of the 3 RCTs were reported in a tailored manner.
Other potential bias
None of the 3 RCTs presented a risk of bias during the meta-analysis. Therefore, the risk was low in terms of other risks of bias.
Primary outcomes
Data on BMD after treatment were reported in the included articles. The measurement method was dual-energy X-ray absorptiometry (DXA) in the studies of McColm [2014], Recknor [2015], and Recker [2015] (13,18,21). We conducted a meta-analysis of 2 doses of blosozumab, namely, 270 mg Q2W and 180 mg Q4W. We found that the BMD data obtained following the 2 drug regimens were highly heterogeneous [heterogeneity: Tau²=2.79; Chi²=11.70, degrees of freedom (df) =1 (P=0.0006); I²=91%], and therefore a meta-analysis was not conducted. The RCT of McColm [2014] showed that both 270 mg Q2W and 180 mg Q4W increased lumbar spine bone density compared with that of the placebo. Comparatively, 270 mg Q2W had a stronger effect on the increase in BMD. However, no BMD data were reported for the hip. The RCT of Recker [2015] showed that both 270 mg Q2W and 180 mg Q4W could improve the BMD of the lumbar spine and femoral neck.
Secondary outcomes
Two RCTs {McColm [2014] and Recknor [2015]} reported changes in OC, BSAP, PINP, and CTX at the end of treatment. Among them, the data of BSAP, PINP, and CTX were highly heterogeneous, and therefore a meta-analysis was not conducted. Heterogeneity was low at 180 mg Q4W, and a fixed-effect model was adopted. The overall results suggested that OC was increased significantly at the end of treatment [heterogeneity: Chi²=0.00, df =1 (P=0.96); I²=0%, test for overall effect: Z =5.64 (P<0.00001)] (Figure 4).
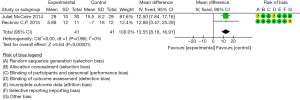
Adverse events
Adverse events were reported in all 3 RCTs {McColm [2014], Recker [2015], and Recknor [2015]}, and the incidence of adverse events did not affect the experimental results.
Discussion
The results of this study showed that the administration of 270 mg Q2W and 180 mg Q4W blosozumab could improve lumbar bone mass in postmenopausal women. Accordingly, the biomarkers PINP, BSAP, OC, and CTX showed significant changes. We did not conduct a meta-analysis of hipbone density because the relevant data were not reported in one of the studies {McColm [2014]}. No significant adverse events were found that could affect the safety of the drugs.
The mean age of patients in each of the studies was different. The mean age of patients in McColm’s [2014] study was 46 years, while that of Recker’s [2015] study was 53 years. The baseline values of lumbar vertebra bone density in the RCTs were also different. The baseline value of McColm’s study [2014] (T-score) was −1.4 and that of Recker’s study [2015] (T-score) was −2.8. These differences affected the applicability of the results. Furthermore, there was a difference in the study population. The population of McColm’s study [2014] comprised Europeans, while that of Recker’s study [2015] included Americans. Therefore, while this meta-analysis data are applicable to European and American populations, they may not be applicable to other areas.
Sclerosin is a protein produced by osteoblasts that is present on the surface of bone cells. It consists of 213 amino acids (22). The main role of sclerosin is to inhibit the differentiation of osteoblasts and indirectly promote the formation of osteoclasts by enhancing the Wnt signaling pathway (23,24). Because of these functions, it has a reverse inhibitory effect on bone formation. In 2015, Yorgan proved that sclerosin binds to low-density lipoprotein receptor-related protein 5 (LRP5) in vivo using transgenic experiments (25). It has been reported that decreased expression of SOST and LRP5 promotes bone mass increase and that SOST could target and bind to LRP5/6, thereby inhibiting the Wnt pathway (26). There are several signaling pathways involved in bone remodeling, but the Wnt signaling pathway plays a key role. The canonical Wnt signaling pathway normally functions as follows. The receptor receives signals, inhibits glycogen synthase kinase-3 beta (GSK-3β), and reduces the phosphorylation of β-catenin, whose level increases in the cytoplasm. Thereafter, β-catenin enters the nucleus to activate the transcription of target genes, promoting the differentiation of osteoblasts, and thereby promoting the generation of bone. Sclerosin can bind directly to the LRP receptor, inhibiting the Wnt signaling pathway, resulting in the ubiquitination of β-catenin, which ultimately leads to proteasomal degradation of β-catenin and the termination of signal transmission. Consequently, osteoblast differentiation is inhibited (27).
Sclerosin can also stimulate osteoclast differentiation through the RANKL pathway, which in turn leads to bone resorption. Therefore, the effect of sclerosin on bone is negative. Synthetic monoclonal antibodies can precisely inhibit sclerosin and promote bone formation. a study has shown that sclerosin, secreted from bone cells, increases with long-term bone disuse and that bone formation during parathyroid therapy is associated with the inhibition of sclerosin, suggesting that teriparatide also has an inhibitory effect on sclerosin (28).
In animal experiments, sclerosin-antibody (scl-ab) improved the bone mass in castrated rats, and the formation of bone cortex and cancellous bone was significantly increased, which led to an increase in bone trabeculae and the thickening of bone cortex (29). The activity and number of osteoblasts also increased in these experiments. The osteogenic effect of scl-ab was not affected by bone turnover, bone resorption, and bisphosphate treatment. Furthermore, bone resorption was observed regardless of the degree of bone formation, and scl-ab had no effect on bone matrix mineralization. In a study on Macaca fascicularis, it was found that the osteogenesis of bone trabeculae and periosteum was positively correlated with the dose of scl-ab injection (30).
Significant research has been conducted at the genetic level. Richards screened 8,557 patients for 2 variants associated with BMD in the lumbar spine and femoral neck, and the LRP5 and TNFRSF11B (OPG) position variants were analyzed. Moreover, Styrkarsdottir screened 6,865 Icelanders and found that the TNFRSF11B (OPG) variant was indeed associated with BMD and osteoporotic fractures. With subsequent expansion of the sample size, other variations of TFNRSF11A (RANK), SOST, microtubule affinity regulating kinase 3 (MARK3), and SP7 were found (31). Furthermore, Wnt16 has been found to be associated with variations in bone density in the skulls of children and the whole body. Subsequent meta-analysis of genes revealed 13 osteoporosis-related variants (32), and 14 BMD-related genes were found in the Genetic Factors for Osteoporosis Consortium (GEFOS) meta-analysis, including family with sequence similarity 210 member A (FAM210A), solute carrier family 25 member 13 (SLC25A13), LRP5, matrix extracellular phosphoglycoprotein (MEPE), spectrin beta, non-erythrocytic 1 (SPTBN1), and dickkopf 1 (DKK1). Moreover, the correlation between osteoporosis and sex chromosome Xp22.31 has been determined (32). With the deepening of research, several gene loci have been discovered; for instance, 36 new mutation loci were discovered by Medina in 2008 (33).
These findings suggested a relationship between common variation sites and BMD, which could explain the variation of 10–20% of bone phenotypes. However, rare variants have a greater effect on BMD than common variants. Whole-genome sequencing of patients with extremely high or low BMD revealed that a nonsense mutation of LGR4 was related to low BMD, and that mutation in collagen type I alpha 2 chain (COL1A2) was closely related to low BMD (34). Natriuretic peptide receptor 3 (NPR3) is associated with high bone density in the lumbar spine, spondin 1 (SPON1) is associated with high hip bone density, and variants of engrailed homeobox 1 (EN1) are closely related to fracture risk (35).
The above findings suggested the role of sclerosin in promoting bone formation and also inhibiting bone resorption. The clinical trial results also confirmed the efficacy of monoclonal antibody in the treatment of osteoporosis, and this strategy of precise treatment of osteoporosis has a far-reaching effect. The guidelines for the treatment of osteoporosis mention that lifestyle management can have a significant effect on the treatment of osteoporosis. With age, muscle mass is lost, which leads to decreased mobility and increased risk of falls and fractures (36). The strengthening effect on bone formation is caused by muscle contraction, and the reduction of muscle also reduces the strengthening effect of low-frequency vibration generated by muscle on the bone, thus making osteoporosis increasingly serious. Therefore, muscle therapy should be used in the treatment of osteoporosis. However, currently, drugs targeting sarcopenia are not approved (37).
The effect of obesity on osteoporosis is also significant. a study has shown that obesity can lead to the accumulation of fat tissue in the bone marrow cavity, resulting in a chronic inflammatory state of the marrow cavity (38). The inflammatory state can enhance the activity of osteoclasts, inhibit the osteogenesis of mesenchymal stem cells to differentiate in the bone marrow, and disrupt the balance of the bone. Therefore, a long-term state of obesity can decrease BMD. One of the main causes of obesity is sedentary muscular dystrophy. According to epidemiological surveys, 40% adults and 20% adolescents in the United States are obese (39). Obesity causes osteoporosis and also chronic inflammation such as osteoarthritis, increasing the risk of tumor development, type 2 diabetes, and cardiovascular diseases (40).
The effect of inflammation on bone should not be underestimated. Firstly, pathogenic stimulus factors, both infectious and noninfectious, activate the innate and special immunity of the body and produce a large number of inflammatory factors to prevent such stimulation from affecting the body. However, cytokines produced by inflammation, such as interferon, interleukin, and chemokines, affect the balance between bone formation and destruction (41). The effects of proinflammatory factors on osteoclasts are mainly manifested in the level of tumor necrosis factor (TNF), interleukin-1 (IL-1), and interleukin-6 (IL-6), which are produced by several inflammatory cells and involve several signaling pathways. They also activate several noninflammatory mediators and proteases to degrade tissues. TNF production is mainly mediated by p55TNF and IL-6 production is mainly mediated by IL-6r and gp130, which activate downstream cytokines and ultimately regulate gene expression (42).
In the process of bone formation, chronic inflammation also has a significant influence on osteoblasts. In rheumatoid arthritis, osteoblasts exist at the site of joint erosion, but their activity and number are not high, which cannot offset the role of bone destruction. The production of the proinflammatory factor TNF is mediated by p55TNF and is inhibited by runt-related transcription factor-2 (RUNX2) ubiquitination (43). IL-6 inhibits the production of osteoblasts and proinflammatory factors and is mediated by DKK1; however, DKK1 is an inhibitor of the Wnt signaling pathway. Sclerosin is an osteoblast activation inhibitor that inhibits the Wnt signaling pathway and antagonizes bone morphogenetic proteins (BMPS) by binding to it (44).
Therefore, the treatment of osteoporosis should involve strengthening the management of obesity, reducing the possibility of inflammation of adipose tissue, and reducing the pathogenic factors of osteoporosis. Studies have shown that weight-bearing exercise is the basis of osteoporosis exercise therapy. According to guidelines from the osteoporosis foundation, exercise should be alternated between high and low intensities and should be performed for at least 30 minutes per day on more than 5 days a week.
The stimulation signals generated by mechanical strain enter the bone cells via the conduction of osteoblasts or the protrusion of bone cells, regulating bone balance. Furthermore, exercise can also reduce body fat content and increase muscle content and bone conversion rate. While treating osteoporosis with drugs, treatment of osteoporosis should be managed in various ways, including muscle exercise, obesity control, and gene therapy.
We presented the first systematic review and meta-analysis of blosozumab in postmenopausal women with reduced bone mass. The RCTs included in the study were assessed for risk by 2 researchers independently, and low risk of bias indicated high quality of the RCTs.
There were some limitations in this meta-analysis. This meta-analysis included only 3 RCTs, although their quality was relatively high. Therefore, in the future, more clinical studies on blosozumab are needed to provide a broader database to promote the use of blosozumab.
The implications of this meta-analysis for clinical practice are as follows. First, current clinical trials have demonstrated that blosozumab can improve lumbar bone density in postmenopausal women. As a monoclonal antibody of sclerosin, blosozumab can act on sclerosin precisely. Inhibition of sclerosin binding to osteoblastic surface receptor LRP5/6 affects the process of osteogenesis. Secondly, there are only a few clinical studies in this field, and therefore more studies should focus on the value of blosozumab in the treatment of osteoporosis, and more clinical studies should be conducted. Finally, the treatment of osteoporosis can be divided into 2 aspects: promoting bone formation and inhibiting absorption. Currently, bisphosphonates are widely used in the treatment of osteoporosis, which mainly inhibit the damage of osteoclasts to the bone and have no obvious effect on the formation of bone. Blosozumab is the latest drug to promote bone formation, which is currently in clinical trials. The results of clinical trials showed that blosozumab can promote improvement in bone density, suggesting that this drug could have a significant effect on the treatment of osteoporosis in the future.
Acknowledgments
Funding: This work was supported by Shandong Provincial Natural Science Foundation (No. ZR2021MH071, to Liang Ma), China Postdoctoral Science Foundation (Nos. 2020M682220 and 2021T140423, to Liang Ma), Shandong Province Key R&D Program (Major Technological Innovation Project) (No. 2021CXGC010501, to Gang Li).
Footnote
Reporting Checklist: The authors have completed the PRISMA reporting checklist. Available at https://apm.amegroups.com/article/view/10.21037/apm-22-998/rc
Conflicts of Interest: All authors have completed the ICMJE uniform disclosure form (available at https://apm.amegroups.com/article/view/10.21037/apm-22-998/coif). GL reports the funding from Shandong Province Key R&D Program (Major Technological Innovation Project) (No. 2021CXGC010501). LM reports funding from Shandong Provincial Natural Science Foundation (No. ZR2021MH071) and China Postdoctoral Science Foundation (Nos. 2020M682220 and 2021T140423). The other authors have no conflicts of interest to declare.
Ethical Statement:
Open Access Statement: This is an Open Access article distributed in accordance with the Creative Commons Attribution-NonCommercial-NoDerivs 4.0 International License (CC BY-NC-ND 4.0), which permits the non-commercial replication and distribution of the article with the strict proviso that no changes or edits are made and the original work is properly cited (including links to both the formal publication through the relevant DOI and the license). See: https://creativecommons.org/licenses/by-nc-nd/4.0/.
References
- Zhu X, Bai W, Zheng H. Twelve years of GWAS discoveries for osteoporosis and related traits: advances, challenges and applications. Bone Res 2021;9:23. [Crossref] [PubMed]
- Zdzieblik D, Oesser S, König D. Specific Bioactive Collagen Peptides in Osteopenia and Osteoporosis: Long-Term Observation in Postmenopausal Women. J Bone Metab 2021;28:207-13. [Crossref] [PubMed]
- Consensus development conference. prophylaxis and treatment of osteoporosis. Br Med J (Clin Res Ed) 1987;295:914-5. [Crossref] [PubMed]
- Stattin K, Michaëlsson K, Larsson SC, et al. Leisure-Time Physical Activity and Risk of Fracture: A Cohort Study of 66,940 Men and Women. J Bone Miner Res 2017;32:1599-606. [Crossref] [PubMed]
- Schuit SC, van der Klift M, Weel AE, et al. Fracture incidence and association with bone mineral density in elderly men and women: the Rotterdam Study. Bone 2004;34:195-202. [Crossref] [PubMed]
- Cosman F, de Beur SJ, LeBoff MS, et al. Clinician's Guide to Prevention and Treatment of Osteoporosis. Osteoporos Int 2014;25:2359-81. [Crossref] [PubMed]
- Khosla S, Bilezikian JP, Dempster DW, et al. Benefits and risks of bisphosphonate therapy for osteoporosis. J Clin Endocrinol Metab 2012;97:2272-82. [Crossref] [PubMed]
- Rachner TD, Khosla S, Hofbauer LC. Osteoporosis: now and the future. Lancet 2011;377:1276-87. [Crossref] [PubMed]
- Ettinger B, Black DM, Mitlak BH, et al. Reduction of vertebral fracture risk in postmenopausal women with osteoporosis treated with raloxifene: results from a 3-year randomized clinical trial. Multiple Outcomes of Raloxifene Evaluation (MORE) Investigators. JAMA 1999;282:637-45. [Crossref] [PubMed]
- Neer RM, Arnaud CD, Zanchetta JR, et al. Effect of parathyroid hormone (1-34) on fractures and bone mineral density in postmenopausal women with osteoporosis. N Engl J Med 2001;344:1434-41. [Crossref] [PubMed]
- Miller PD, Hattersley G, Riis BJ, et al. Effect of Abaloparatide vs Placebo on New Vertebral Fractures in Postmenopausal Women With Osteoporosis: A Randomized Clinical Trial. JAMA 2016;316:722-33. [Crossref] [PubMed]
- Saag KG, Petersen J, Brandi ML, et al. Romosozumab or Alendronate for Fracture Prevention in Women with Osteoporosis. N Engl J Med 2017;377:1417-27. [Crossref] [PubMed]
- McColm J, Hu L, Womack T, et al. Single- and multiple-dose randomized studies of blosozumab, a monoclonal antibody against sclerostin, in healthy postmenopausal women. J Bone Miner Res 2014;29:935-43. [Crossref] [PubMed]
- Lewiecki EM. New targets for intervention in the treatment of postmenopausal osteoporosis. Nat Rev Rheumatol 2011;7:631-8. [Crossref] [PubMed]
- Hay E, Bouaziz W, Funck-Brentano T, et al. Sclerostin and Bone Aging: A Mini-Review. Gerontology 2016;62:618-23. [Crossref] [PubMed]
- Li X, Ominsky MS, Warmington KS, et al. Sclerostin antibody treatment increases bone formation, bone mass, and bone strength in a rat model of postmenopausal osteoporosis. J Bone Miner Res 2009;24:578-88. [Crossref] [PubMed]
- Ominsky MS, Vlasseros F, Jolette J, et al. Two doses of sclerostin antibody in cynomolgus monkeys increases bone formation, bone mineral density, and bone strength. J Bone Miner Res 2010;25:948-59. [Crossref] [PubMed]
- Recknor CP, Recker RR, Benson CT, et al. The Effect of Discontinuing Treatment With Blosozumab: Follow-up Results of a Phase 2 Randomized Clinical Trial in Postmenopausal Women With Low Bone Mineral Density. J Bone Miner Res 2015;30:1717-25. [Crossref] [PubMed]
- Page MJ, McKenzie JE, Bossuyt PM, et al. The PRISMA 2020 statement: an updated guideline for reporting systematic reviews. BMJ 2021;372: [PubMed]
- Higgins J, Thomas J. Cochrane handbook for systematic reviews of interventions. John Wiley & Sons, 2019. Available online: https://training.cochrane.org/handbook/archive/v6
- Recker RR, Benson CT, Matsumoto T, et al. A randomized, double-blind phase 2 clinical trial of blosozumab, a sclerostin antibody, in postmenopausal women with low bone mineral density. J Bone Miner Res 2015;30:216-24. [Crossref] [PubMed]
- Balemans W, Van Hul W. Human genetics of SOST. J Musculoskelet Neuronal Interact 2006;6:355-6. [PubMed]
- Wang Y, Li YP, Paulson C, et al. Wnt and the Wnt signaling pathway in bone development and disease. Front Biosci (Landmark Ed) 2014;19:379-407. [Crossref] [PubMed]
- Yorgan TA, Peters S, Jeschke A, et al. The Anti-Osteoanabolic Function of Sclerostin Is Blunted in Mice Carrying a High Bone Mass Mutation of Lrp5. J Bone Miner Res 2015;30:1175-83. [Crossref] [PubMed]
- Semënov M, Tamai K, He X. SOST is a ligand for LRP5/LRP6 and a Wnt signaling inhibitor. J Biol Chem 2005;280:26770-5. [Crossref] [PubMed]
- Johnson ML, Recker RR. Exploiting the WNT Signaling Pathway for Clinical Purposes. Curr Osteoporos Rep 2017;15:153-61. [Crossref] [PubMed]
- Moustafa A, Sugiyama T, Prasad J, et al. Mechanical loading-related changes in osteocyte sclerostin expression in mice are more closely associated with the subsequent osteogenic response than the peak strains engendered. Osteoporos Int 2012;23:1225-34. [Crossref] [PubMed]
- Li X, Ominsky MS, Warmington KS, et al. Increased bone formation and bone mass induced by sclerostin antibody is not affected by pretreatment or cotreatment with alendronate in osteopenic, ovariectomized rats. Endocrinology 2011;152:3312-22. [Crossref] [PubMed]
- Ominsky MS, Niu QT, Li C, et al. Tissue-level mechanisms responsible for the increase in bone formation and bone volume by sclerostin antibody. J Bone Miner Res 2014;29:1424-30. [Crossref] [PubMed]
- Styrkarsdottir U, Halldorsson BV, Gretarsdottir S, et al. New sequence variants associated with bone mineral density. Nat Genet 2009;41:15-7. [Crossref] [PubMed]
- Rivadeneira F, Styrkársdottir U, Estrada K, et al. Twenty bone-mineral-density loci identified by large-scale meta-analysis of genome-wide association studies. Nat Genet 2009;41:1199-206. [Crossref] [PubMed]
- Zheng HF, Forgetta V, Hsu YH, et al. Whole-genome sequencing identifies EN1 as a determinant of bone density and fracture. Nature 2015;526:112-7. [Crossref] [PubMed]
- Styrkarsdottir U, Thorleifsson G, Sulem P, et al. Nonsense mutation in the LGR4 gene is associated with several human diseases and other traits. Nature 2013;497:517-20. [Crossref] [PubMed]
- Trajanoska K, Rivadeneira F. The genetic architecture of osteoporosis and fracture risk. Bone 2019;126:2-10. [Crossref] [PubMed]
- Rosenberg IH. Sarcopenia: origins and clinical relevance. Clin Geriatr Med 2011;27:337-9. [Crossref] [PubMed]
- Black DM, Rosen CJ. Clinical Practice. Postmenopausal Osteoporosis. N Engl J Med 2016;374:254-62. [Crossref] [PubMed]
- Tilg H, Moschen AR. Adipocytokines: mediators linking adipose tissue, inflammation and immunity. Nat Rev Immunol 2006;6:772-83. [Crossref] [PubMed]
- Ogden CL, Carroll MD, Kit BK, et al. Prevalence of childhood and adult obesity in the United States, 2011-2012. JAMA 2014;311:806-14. [Crossref] [PubMed]
- Wearing SC, Hennig EM, Byrne NM, et al. The biomechanics of restricted movement in adult obesity. Obes Rev 2006;7:13-24. [Crossref] [PubMed]
- Redlich K, Smolen JS. Inflammatory bone loss: pathogenesis and therapeutic intervention. Nat Rev Drug Discov 2012;11:234-50. [Crossref] [PubMed]
- Kishimoto T. IL-6: from its discovery to clinical applications. Int Immunol 2010;22:347-52. [Crossref] [PubMed]
- Mukai T, Otsuka F, Otani H, et al. TNF-alpha inhibits BMP-induced osteoblast differentiation through activating SAPK/JNK signaling. Biochem Biophys Res Commun 2007;356:1004-10. [Crossref] [PubMed]
- Li X, Zhang Y, Kang H, et al. Sclerostin binds to LRP5/6 and antagonizes canonical Wnt signaling. J Biol Chem 2005;280:19883-7. [Crossref] [PubMed]
- Pagnotti GM, Styner M, Uzer G, et al. Combating osteoporosis and obesity with exercise: leveraging cell mechanosensitivity. Nat Rev Endocrinol 2019;15:339-55. [Crossref] [PubMed]