Network pharmacology-based prediction of inhibiting leukocyte recruitment and angiogenesis of total glucosides of peony against rheumatoid arthritis
Introduction
Rheumatoid arthritis (RA) is a chronic autoimmune disease characterized by inflammatory changes in the synovial tissues, cartilage, and bones of the joints, ultimately leading to disability and death. The incidence of RA is on the rise, which poses a serious threat to health and quality of life (1). Steroidal anti-inflammatory drugs (glucocorticoids), nonsteroidal anti-inflammatory drugs, immunosuppressive drugs (chemical drugs and biological drugs), and Chinese natural medicines are applied to treat RA in the clinic. It requires chronic, even life-long treatments, so the safety of treatments is gaining attention. Recently, the development of natural products has attracted attention (2).
Total glucosides of peony (TGP) is extracted from Paeonia lactiflora Pallas (3). TGP capsules (Pavlin) have been approved for RA treatment since 1998. It is also used for systemic lupus erythematosus, Sjögren syndrome, psoriasis, ankylosing spondylitis, immune liver injury treatment in China (4-8). It could improve the clinical effects and reduce toxicity when used with methotrexate and leflunomide (9). Numerous studies have confirmed the anti-inflammatory and immunomodulatory effects of TGP. It could inhibit B cell activation, regulate macrophage polarization, prevent dendritic cells (DCs) from maturation and inhibit the abnormal proliferation of synoviocytes (3,10,11). Although research on the mechanisms of TGP for RA treatment has continued, no systematic and comprehensive understanding of the relationships between targets and pathways involved in the treatment has emerged. Otherwise, there are fifteen monoterpene glycosides, such as paeoniflorin (PF), albiflorin (AF) and oxypaeoniflorin (Oxy-PF) contained in TGP (1). Previous researches focused largely on the effects of PF, the major ingredient of TGP. The functions of other monoterpene glycosides have not been clarified.
Network pharmacology has been one of the new strategies for multi-target drug discovery based on bioinformatics and systems biology (12-14). In this study, we investigated the comprehensive mechanism of TGP for RA treatment using network pharmacology analysis (Figure 1). We analyzed the different targets of the components in TGP. We found the new effects of TGP on inhibiting leukocyte recruitment and angiogenesis. This provides a better understanding of TGP on the RA treatment.
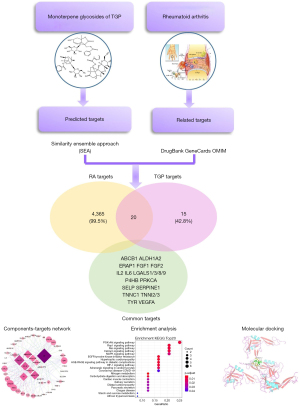
Methods
Screen the included components
The study was conducted in accordance with the Declaration of Helsinki (as revised in 2013). The web of science, PubMed, CNKI Databases were searched to acquire information on TGP with the keywords “Total glucosides of peony”, “TGP”, “Paeonia lactiflora Pall”. Traditional Chinese Medicine Systems Pharmacology Database (TCMSP, https://old.tcmsp-e.com/tcmsp.php) (15) and Chinese Pharmacopoeia, relevant textbooks, reviews, and documents were also consulted for complete information.
Identify the potential targets of TGP components
There are four kinds of target-prediction methods: based on chemical similarity, molecular docking, machine learning and a combined forecast (16). In our study, the targets prediction was conducted on the Similarity Ensemble Approach (http://sea.bkslab.org/) based on chemical similarity (16). The simplified molecular-input line-entry system (SMILES) codes of the components in were gotten from Pubchem (listed in Table 1), and then were submitted to the Similarity Ensemble Approach server for target prediction based on chemical similarity. Predicted targets with P values were generated.
Table 1
PubChem CID | Components | Molecular formula | Molecular weight | Molecular structure | SMILEs |
---|---|---|---|---|---|
442534 | Paeoniflorin | C23H28O11 | 480.5 | ![]() |
CC12CC3(C4CC1(C4(C(O2)O3)COC(=O)C5=CC=CC=C5)OC6C(C(C(C(O6)CO)O)O)O)O |
21631105 | Oxy-paeoniflorin | C23H28O12 | 496.5 | ![]() |
CC12CC3(C4CC1(C4(C(O2)O3)COC(=O)C5=CC=C(C=C5)O)OC6C(C(C(C(O6)CO)O)O)O)O |
21631106 | Benzoyl-paeoniflorin | C30H32O12 | 584.6 | ![]() |
CC12CC3(C4CC1(C4(C(O2)O3)COC(=O)C5=CC=CC=C5)OC6C(C(C(C(O6)COC(=O)C7=CC=CC=C7)O)O)O)O |
13811386 | Benzoyloxy-peoniflorin | C30H32O13 | 600.6 | ![]() |
CC12CC3(C4CC1(C4(C(O2)O3)COC(=O)C5=CC=C(C=C5)O)OC6C(C(C(C(O6)COC(=O)C7=CC=CC=C7)O)O)O)O |
494717 | Galloyl-paeoniflorin | C30H32O15 | 632.6 | ![]() |
CC12CC3(C4CC1(C4(C(O2)O3)COC(=O)C5=CC=CC=C5)OC6C(C(C(C(O6)COC(=O)C7=CC(=C(C(=C7)O)O)O)O)O)O)O |
101382399 | Paeoniflorin sulfonate | C23H28O14S | 560.5 | ![]() |
CC12CC3(C4CC1(C4(C(O2)O3)COC(=O)C5=CC=CC=C5)OC6C(C(C(C(O6)CO)O)O)O)OS(=O)(=O)O |
102516499 | 3'-O-Galloyl-paeoniflorin | C30H32O15 | 632.6 | ![]() |
CC12CC3(C4CC1(C4(C(O2)O3)COC(=O)C5=CC=CC=C5)OC6C(C(C(C(O6)CO)O)OC(=O)C7=CC(=C(C(=C7)O)O)O)O)O |
137705343 | Debenzoylgalloyl-paeoniflorin | C23H28O14 | 528.5 | ![]() |
CC12CC3(C4CC1(C4(C(O2)O3)CO)OC5C(C(C(C(O5)COC(=O)C6=CC(=C(C(=C6)O)O)O)O)O)O)O |
71452334 | Paeoniflorin B | C36H42O17 | 746.7 | ![]() |
CC12CC3(C4CC1(C4(C(O2)O3)COC(=O)C5=CC=CC=C5)OC6C(C(C(C(O6)COC(=O)C7=CC=CC=C7)OC8C(C(C(C(O8)CO)O)O)O)O)O)O |
51346141 | Albiflorin | C23H28O11 | 480.5 | ![]() |
CC12CC(C3CC1(C3(C(=O)O2)COC(=O)C4=CC=CC=C4)OC5C(C(C(C(O5)CO)O)O)O)O |
50163461 | Albiflorin R1 | C23H28O11 | 480.5 | ![]() |
CC12CC(C3CC1(C3(C(=O)O2)COC(=O)C4=CC=CC=C4)OC5C(C(C(C(O5)CO)O)O)O)O |
102000323 | Benzoyl-albiflorin | C30H32O12 | 584.6 | ![]() |
CC12CC(C3CC1(C3(C(=O)O2)COC(=O)C4=CC=CC=C4)OC5C(C(C(C(O5)COC(=O)C6=CC=CC=C6)O)O)O)O |
138108175 | 4-O-Galloyl-albiflorin | C30H32O15 | 632.6 | ![]() |
CC12CC(C3CC1(C3(C(=O)O2)COC(=O)C4=CC=CC=C4)OC5C(C(C(C(O5)CO)O)O)O)OC(=O)C6=CC(=C(C(=C6)O)O)O |
124079396 | 6'-O-Galloyl-albiflorin | C30H32O15 | 632.6 | ![]() |
CC12CC(C3CC1(C3(C(=O)O2)COC(=O)C4=CC=CC=C4)OC5C(C(C(C(O5)COC(=O)C6=CC(=C(C(=C6)O)O)O)O)O)O)O |
14605198 | Lactiflorin | C23H26O10 | 462.4 | ![]() |
CC12C3(CC4C3(C(O1)CC4=O)COC(=O)C5=CC=CC=C5)OC6C(O2)C(C(C(O6)CO)O)O |
TGP, total glucosides of peony; SMILES, simplified molecular-input line-entry system.
The molecular mechanism of RA
The molecular related with the occurrence and development of RA was obtained from four sources: DrugBank (http://www.drugbank.ca/, version 4.3) (17), the GeneCards database (https://www.genecards.org/) (18), DisGeNET databases (https://www.disgenet.org/) (19) and the Online Mendelian Inheritance in Man (OMIM) database (http://www.omim.org/, updated on April 5, 2021) (20). The Gene ontology (GO) and the Kyoto Encyclopedia of Genes and Genomes (KEGG) enrich functions of these molecular were further analyzed in CTD database (http://ctdbase.org/tools/analyzer.go) (21).
Construct components-targets networks
Cytoscape 3.6.1 is an open-source bioinformatics software platform that helps visualize molecular interaction networks. The “Network Analyzer” plug-in was used to calculate the topology parameters of the network.
Enrichment analysis
Once we identified the targets of TGP for RA treatment, GO and KEGG functional annotations were carried out according to gene functions. Enrichment analysis was performed with R (version 3.6.0 for Windows). The KEGG database analyzes the pathways and the functions of these targets (22). Pathways were ranked according to the number of molecules in pathways and networks with a cut-off value (P<0.05) for significantly enriched pathways/networks.
Computational validation of components—targets interactions
The hub targets got from network analysis were further ascertain the interactions between active components. The crystal structure of these herb targets and components were obtained from the Research Collaboratory for Structural Bioinformatics (RCBS)-Protein Data Bank database (23). The structures of TGP components and targets proteins were optimized at AutoDockTools-1.5.6 based on least energy. The docking exercise was calculated through Autodock Vina software package (24) and the docking results were showed with PyMol (version 1.1) (25).
Results
The included components of TGP
TGP was extracted from the roots of Paeonia lactiflora Alba with clarification, organic solvent extraction and macroporous resin purification. There are a large amount of tannins, carbohydrate and more than 80 small molecular organic matters contained in the crude Paeonia lactiflora Alba extract (Figure 2A) (26). Approximately 15 monoterpene glycosides have been identified in TGP (Table 1), such as PF, AF, and their derivatives (Figure 2A) (27). PF accounts for over or equal to 40% (28). The quality and reproducibility of TGP extracted from the dried root is controlled by measuring the percentage of PF and AF, which the content should not be less than 40% and 10%, respectively (27). Clinical used TGP capsule (Pavlin) was required not to be less than 300 mg and the PF content should not be less than 104 mg (29).
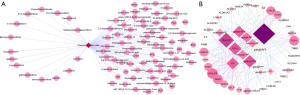
Identify potential targets of TGP and construct the compounds-targets
Targets prediction was conducted on the Similarity Ensemble Approach. As AF and AF-R1 share same SMILES, we predicted the targets of 14 compounds except AF-R1. Figure 2B showed the compounds-targets network of TGP. The diamonds represent compounds; round nodes represent putative targets. The size will increase and the color darkens with increasing degree. There were 49 nodes and 161 edges in TGP compounds-targets network. The average degree was 7.23. 35 kinds of proteins were predicted as the putative targets of TGP and the top 10 were galectin-9 (LGALS9), tyrosyl-DNA phosphodiesterase 1 (TDP1), myelin-associated glycoprotein (MAG), galectin-1 (LGALS1), galectin-3 (LGALS3), fibroblast growth factor 2 (FGF2), fibroblast growth factor 1 (FGF1), vascular endothelial growth factor A (VEGFA), P-selectin (SELP) and multidrug resistance protein 1 (ABCB1) with higher degree values. These targets might be effective on the treatment of RA. PF, Oxy-PF, galloylpaeoniflorin (galloyl-PF3), PF-B, benzoylpaeoniflorin (benzoyl-PF), AF and 4-O-galloylalbiflorin (galloyl-AF4) were the major active compounds with more predicted targets. More than 50% of the predicted targets were common in these monoterpene glycosides, implying their synergistic effects.
PF and AF are the major components of TGP, the component-target network of the two components and their derivatives were analyzed separately (Figure 3A,3B). The major predicted targets of PF and its derivants were MAG, LGALS1, TDP1, LGALS3, FGF1, FGF2, LGALS9, VEGFA and SELP, while that were LGALS9, TDP1, ABCB1, AMY1A, FGF1, FGF2, LGALS1, LGALS3, protein kinase C alpha type (PRKCA) and VEGFA for AF and its derivants in degree orders (Figure 3A,3B). It should be noted that AF and its derivatives have more kinds of targets (35 kinds) than PF and its derivatives (29 kinds). The five unique targets of AF and its derivants were carbonic anhydrase 12 (CA12), carbonic anhydrase 9 (CA9), IL-6, Ras guanyl-releasing protein 3 (RASGRP3), and sodium/glucose cotransporter 4 (SLC5A4), which may show their differences in the therapeutic effects and adverse reactions.
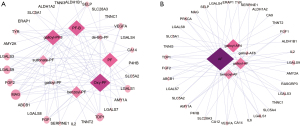
The affinity score of each component with their predicted targets was ranged from 0.28 to 0.46 (Figure 4). Fifty percent of the putative targets of PF and AF were common, implying a close relationship between the two components. Debenzoylgalloyl-paeoniflorin, 6'-O-Galloyl-albiflorin and galloyl-paeoniflorin have high affinity with the TDP1 and SERPINE1, but their targets were not common to the major targets of TGP. Lactiflorin (LF) was reported to exist in the amounts of 0.35–0.64% in Paeoniae Radix with high performance liquid chromatography (HPLC) analysis, which was much less than PF (30). So far, there were no reported derivants of LF in TGP. The predicted targets of LF were SELP, PRKCA, LGALS9, LGALS7, LGALS3, LGALS1 and ABCB1, which were similar as PF and AF for chemical similarity.
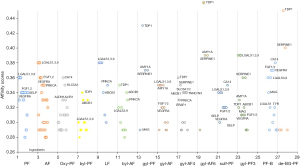
Identify genes and proteins associated with RA
A total of 3,523 known protein-coding genes for RA were collected from the GeneCards database. Two hundred and ninety-six and 2,722 protein-coding genes for RA were obtained from the OMIM and DisGeNET databases, respectively. One hundred and twenty-four therapeutic targets for RA treatment were obtained from the TTD database. After elimination of redundancies and duplicate data, 4,750 symbols for RA were obtained (available online: https://cdn.amegroups.cn/static/public/apm-21-2203-1.xlsx). After removing the microRNA and the pseudogene, 4,385 symbols for RA were obtained. These biomarkers could be enriched into 940 KEGG pathways and their functions involving innate immune system, adaptive immune system, signaling by interleukins, metabolism, bone destruction, angiogenesis, and tissue remodeling, different manners of cell death, cell proliferation, cell differentiation, neurosensory function and signal transduction. The main function pathways were GPCR downstream signaling, signaling by insulin receptor, JAK-STAT signaling pathway, T cell receptor signaling pathway, toll-like receptor signaling pathway, IL-17 signaling pathway, Interleukin-6 signaling, NF-kappa B signaling pathway, estrogen signaling pathway, B cell receptor signaling pathway, Wnt signaling pathway, MyD88 pathways, and so on. The functions of Th17 cell differentiation, activation of B and T cells, natural killer cell mediated cytotoxicity, Th1 and Th2 cell differentiation, platelet activation, activation of C3/C5 and homeostasis, cellular response to hypoxia, the migration of neutrophils also play important roles in the RA processing (available online: https://cdn.amegroups.cn/static/public/apm-21-2203-2.xlsx).
Identify the targets of TGP relevant to the RA treatment
Venn diagram was drawn to perform intersection alignment with the TGP targets (Figure 5A). There were 20 coincidence targets predicted for RA treatment including ABCB1, mitochondrial aldehyde dehydrogenase X (ALDH1A2), endoplasmic reticulum aminopeptidase 1 (ERAP1), FGF1, FGF2, IL2, IL6, LGALS1/3/8/9, protein disulfide-isomerase (P4HB), PRKCA, SELP, plasminogen activator inhibitor 1 (SERPINE1), troponin C (TNNC1), troponin I 2/3 (TNNI2/3), tyrosinase (TYR) and VEGFA. Some of these targets were also the high degree ones among the TGP predicted.
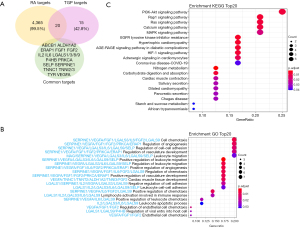
Enrichment of the GO functions and KEGG pathways
To investigate the role of the predicted targets, we conducted enrichment of GO pathways to clarify the relevant biologic processes (P<0.01) (Figure 5B). For a brief demonstration, we intercepted the top 20 terms. The results indicated that numerous biologic processes were involved in RA treatment, including cell chemotaxis, the regulation of angiogenesis, cell-cell development, vasculature development, leukocyte migration, and so on. The mainly functions concentrate on the aspect of regulation of angiogenesis, regulation the leukocyte and the tissue remodeling. LGALS3/9, VEGFA, FGF1, SELP, IL-6 and IL-2 of TGP play critical roles in leukocyte traffic progress; VEGFA, FGF1, FGF2, PRKCA and ERAP1 proteins have important roles in anti-angiogenesis (Figure 5C). The enriched KEGG pathways including VEGFR pathway, interleukins signaling, PI3K-Akt pathway, platelet activation, extracellular matrix organization, and so on (Figure 6).
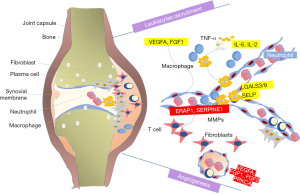
Docking exercises of hub targets and the major components
To ascertain the interaction between active components and their protein targets, computational docking exercises were conducted to mimic the binding characteristics. Here, the components of AF, PF and LF were selected for verification of molecular docking. The targets related to angiogenesis and leukocyte traffic progress were selected.
It is generally accepted that the binding energy value less than -4.25 kcal/mol indicates a certain binding activity, less than −5.0 kcal/mol indicates good binding activity, and less than −7.0 kcal/mol indicates strong binding activity. The binding energy of the AF, PF and LF with these hub targets was shown in Table 2. Binding affinities to these targets distributed within the range of −5.9 to −8.8 kcal/mol, indicating a good or strong binding activity. Docking results were visualized in the Pymol software. Figure 7 showed the three components docking with LGALS1/3/9 and SELP, which are mainly related to the leukocyte traffic progress; Figure 8 shows the three components docking with VEGFA, FGF1 and FGF2, which possess the function of angiogenesis.
Table 2
Targets | PDB ID | Docking with AF (kcal/mol) | Docking with PF (kcal/mol) | Docking with LF (kcal/mol) |
---|---|---|---|---|
LGALS3 | 3ZSM | −7.4 | −6.9 | −7.8 |
LGALS9 | 3NV1 | −6.8 | −6.9 | −7.3 |
LGALS1 | 1GZW | −5.9 | −6.8 | −6.6 |
VEGFA | 1VPF | −6.6 | −6.9 | −7.9 |
FGF1 | 4YOL | −7.8 | −8.2 | −8.2 |
FGF2 | 2BFH | −6.4 | −6.6 | −7.3 |
SELP | 1G1Q | −6.9 | −7.2 | −7.2 |
PRKCA | 4DNL | −6.5 | −7.1 | −8.1 |
ERAP1 | 2YD0 | −8.8 | – | – |
IL-6 | 1ALU | −6.5 | – | – |
IL-2 | 1M47 | −6.3 | – | – |
TGP, total glucosides of peony; AF, albiflorin; PF, paeoniflorin; LF, lactiflorin.
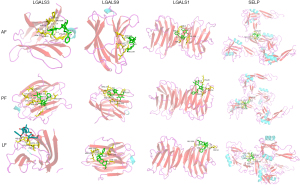
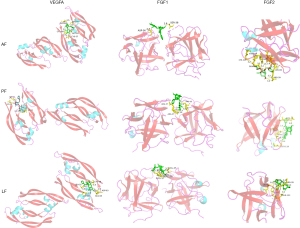
Figure 9 showed the docking results of unique targets of AF involving the leukocyte traffic and angiogenesis progress.
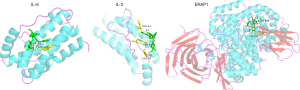
Discussion
TGP has been widely used in China to treat RA through balancing proinflammatory cytokines and anti-inflammatory cytokines and regulating immune cells. In a 24-week, open label, randomized multicenter clinical trial, more patients in TGP group achieved a European League Against Rheumatism (EULAR) good response or moderate response at 12 and 24 weeks (31). TGP can decrease the population of IFN-γ, IL-17-producing cells in RA patients (9). While, several issues need to be studied, such as how these constituents interact with each other, what is the most important component in TGP, whether these components share similar targets, and so on. Therefore, we investigated the comprehensive mechanism by which TGP acts in RA using network pharmacology.
There are 15 monoterpene glycosides identified in TGP. PF and AF are the major contents, which account for more than half of TGP. The other components are the derivatives of PF and AF except the LF. There were no LF associated derivatives. The effects of PF have been well explored, and found that PF is able to help diminish joint pain, reduce joint swelling, inhibit synovial hypertrophy, alleviate the damage of cartilage and bone erosion in experimental arthritis (10). So far, AF is reported to inhibit lung inflammation in asthmatic mice, alleviate high fat, decreased the cholesterol gallstone formation, alleviate neuropathic pain and promote the recovery of bone marrow hemopoietic function (32,33). However, what the functions of AF are in the clinical RA treatment, nobody is carrying out research on it. In our analysis, we found that AF and its derivatives have more kinds of targets than PF and its derivatives. They shared the common targets such as ABCB1, ALDH1A2, ALDH1B1, AMY1A, AMY2A, CA14, ERAP1, FGF1, FGF2, IL2, LGALS1/3/4/7/8/9, MAG, P4HB, SELP, SERPINE1, SLC28A3, SLC5A1/2, SLC5A2, TDP1, TNNC1, TNNI3, TNNT2, TYR and VEGFA, but AF and its derivatives have five unique targets, CA12, CA9, IL-6, RASGRP3 and SLC5A4. This may influence their therapeutic effects and adverse reactions. We should pay attention that only AF and its derivatives target the key cytokine IL-6, which means that the significant role of AF and its derivatives should not be disregarded in RA treatment. Another component worth further investigating is LF, as no reports on its functions so far. In our analysis, we found LF shared the common targets, such as SELP, PRKCA, LGALS9, LGALS3, LGALS1 and ABCB1 with AF and PF. Nevertheless, the level of LF in TGP is very low and the reports on the effects of LF are less; how it affects the therapeutic effects of TGP should be further researched.
Previous researches focused on the effects of TGP on immune cells (T, B-lymphocytes, macrophages, dendritic cells, synoviocytes, endothelial cells, etc.). In our analysis, targets of TGP for RA treatment were ABCB1, ALDH1A2, ERAP1, FGF1, FGF2, IL2, IL6, LGALS1/3/8/9, P4HB, PRKCA, SELP, SERPINE1, TNNC1, TNNI2/3, TYR and VEGFA. Biologic processes of TGP were enriched in cell chemotaxis, the regulation of angiogenesis, cell-cell development, vasculature development, leukocyte migration. The regulation of leukocyte migration and angiogenesis causes our interest as they were not well been reported.
Aberrant recruitment and activation of leukocytes were the special character of RA (34). Leukocytes migrating out of the blood, across the endothelium and through inflamed tissues, further causes a cascade of pathological process, especially in the disease early stage (35). In patients with early RA, a substantial number of leukocytes accumulate under the synovium, and the degree of infiltration correlating with disease activity rather than symptom duration (36). Limiting pathogenic leukocytes trafficking into the joint is possible a new treatment. In our analysis, the predicted targets LGALS3/9, VEGFA, FGF1, IL-6, IL-2 and SELP involve in the regulation of leukocyte recruitment, migration and apoptosis. LGALS3 and LGALS9 belong to a family of lectins, which have been linked to important functions such as differentiation, migration, neutrophil activation and intracellular trafficking (37,38). These proteins are highly expressed in neutrophils (37-39). Galectin-3 was reported involved in the activation, adhesion, and apoptosis of neutrophils (40). By increasing disulfide reductase activity at the plasma membrane, galectin 9 alters the plasma membrane redox state and enhances cell migration (41). Galectin 9 also induces cytokine (IL-6, IL-8, IL-12) and chemokine (CCL2) production (39,42,43), by which control the leukocytes trafficking (44,45). VEGFA induces proliferation and migration of vascular endothelial cells, and is essential for angiogenesis. This protein could promote inflammation by facilitating recruitment of inflammatory cells. SELP is one of important cell adhesion molecules, which have roles in cell proliferation, differentiation, motility, trafficking, apoptosis and tissue architecture (46). SELP mediates the rapid rolling of leukocyte rolling over vascular surfaces and also mediates the interaction of activated endothelial cells or platelets with leukocytes with the help of VEGFA (47). Most components in TGP could bind to these targets, meaning a significant regulation of leukocytes accumulating. This mechanism of TGP on RA treatment has not ever been reported so far.
Uncontrolled neovascularization plays a critical role in joint edema and osteoclastic bone erosion (48). Inhibit the angiogenesis process could ameliorated CIA joint vascularization, swelling and bone destruction (49). In our analysis, the predicted targets VEGFA, FGF1, FGF2, SERPINE1, PRKCA and ERAP1 involve in the TGP’s angiogenesis regulation. It is well known that VEGF-A and FGF2 are the key regulators of angiogenesis (50). VEGF and FGF2 binding to their receptors on endothelial cells starts the angiogenesis process (51). VEGF-A promotes angiogenesis through many mechanisms, including activating the endothelial cells, increasing vascular permeability activity, and promoting cell migration of endothelial cells (52). FGF2 could promote the proliferation, migration and vascular tube formation of endothelial cell apoptosis (53). Activated RA macrophages and synovial tissue fibroblasts induce the expression and secretion of VEGFA and FGF2, and further trigger angiogenesis (54). In experimental arthritis models, anti-VEGF antibody delayed the collagen induced arthritis onset, joint swelling and vascularization (55). TNF, IL-1β, IL-6 inhibitors and Cox-2 inhibitors may function through inhibiting angiogenesis (51-53). PRKCA has been reported to play roles in cell adhesion and transformation (56). SERPINE1, the specific inhibitor of urokinase-type plasminogen activator (uPA) also is recognized to play a vital role in angiogenesis by promoting the proliferation of vascular endothelial cells (57). ERAP1 is involved in the final trimming of peptides within the endoplasmic reticulum for presentation by major histocompatibility complex (MHC) class I molecules, which was found to be associated with ankylosing spondylitis in a genome-wide association study of nonsynonymous single nucleotide polymorphisms (58). The role of ERAP1 in angiogenesis and migration in tumor has attracted attention recently, but no such association has ever been reported in RA (59). VEGFA, FGF1, FGF2, SERPINE1, PRKCA and ERAP1 were the predicted targets of TGP, meaning a significant regulation of angiogenesis through which TGP exerts therapeutic effect on RA. Current study of TGP is focusing on the tissue of anti-inflammatory, immunoregulation and analgesic effects, and the study of angiogenesis was poor reported. The anti-angiogenic effect of Paeonia lactiflora Pallas in the retina of diabetic retinal microvasculopathy has been proved through murine model (60). Previous reports about the regulation of PF on angiogenetic effects were opposite in different diseases. PF performed the pro-angiogenetic effect in vitro and accelerating wound healing in vivo (61), while attenuated the in vitro angiogenesis in oxidized low density lipoprotein-induced human umbilical vein endothelial cells (HUVECs) by inhibiting the VEGF/VEGFR2 (62). However, PF shows angiogenic actions on endothelial progenitor cells of ischemic stroke rat model by increasing the secretion of pro-angiogenic factors (63). The effects of PF on the angiogenesis of RA especially in the inflammatory infiltration environment need further researches. The angiogenesis effects of other components in TGP have not been researched.
Patients with RA experience a higher rate of cardiovascular disease (CVD) events compared with controls. Chronic inflammation contributes to this risk and cumulative inflammation predicted coronary plaque progression in RA (64). Overexpression and secretion of galectin-1, galectin-3 and galectin-9 are associated with heart failure and atherosclerosis. Galectin-1 and galectin-9 were proved to be the biomarkers of endothelial activation and dysfunction in rare systemic autoimmune diseases, which are implications for cardiovascular risk (65). It has been well reported about the contribution of inflammatory cytokines IL-6 and IL-2 to the progression of left ventricular dysfunction, myocardial damage and chronic heart failure syndrome (64-66). FGF1, FGF2 and VEGFA are the vascular growth factors. High concentrations of VEGFA, FGF1 and FGF2 have been found in CVD patients with an unfavorable prognosis and disease severity (67). The relationship between VEGFA and the heart is double-sided. VEGFA activates cardiomyocytes, further promotes the morphogenesis, contractility and wound healing. However, it is also associates with inflammation, mechanical stress and cytokine stimulation in the CVD (68). Therefore, these main predicted targets of TGP remind us the cardiovascular protective effects of TGP on RA treatment. Previous research had demonstrated the myocardial protective effects of TPG in both isoprenaline-induced myocardial ischemia rat and acute myocardial infarction rat (69,70). TGP also suppressed myocardial remodeling by regulating the NF-κB pathway (71). The effects of TGP on CVD protection in RA patients lack of clinical evidence in RA, which deserves further investigation.
Our research firstly predicted the inhibiting of leukocyte migration and angiogenesis of TGP. These predictions revealed new treatment for RA. However, certain limitations existed in our study. Firstly, more methods and databases should be used for targets prediction to get more related targets. Second, due to the difficulty of data acquisition, the database may not include all known or unknown targets and protein-protein interactions. This situation could be improved in the future when more data were available. If possible, the differential gene expression in patients only receiving TGP treatment should be detected to prove the predicted targets. However, there were not enough patients to meet the criteria so far. Further clinical and experimental validation is needed.
Conclusions
In the present study, we revealed 20 potential targets of 14 monoterpene glycosides in TGP, and predicted these targets to ameliorate RA through inhibiting leukocyte recruitment and angiogenesis. The role of these targets in CVD events also reminds the possible CVD protection effects of TGP in RA patients. Our research helped to illustrate the mechanisms involved in the action of TGP and may provide a better understanding of its anti-RA effects in terms of inhibiting leukocyte recruitment and angiogenesis.
Acknowledgments
We would like to thank Prof. Minglin Liu for his help in polishing our paper.
Funding: This study was funded by National Natural Science Foundation of China (No. 81903934) and Tianjin Health Science and Technology Project (No. ZC20205).
Footnote
Conflicts of Interest: All authors have completed the ICMJE uniform disclosure form (available at https://apm.amegroups.com/article/view/10.21037/apm-21-2203/coif). All authors report receiving funding support from the National Natural Science Foundation of China (No. 81903934) and Tianjin Health Science and Technology Project (No. ZC20205). The authors have no other conflicts of interest to declare.
Ethical Statement:
Open Access Statement: This is an Open Access article distributed in accordance with the Creative Commons Attribution-NonCommercial-NoDerivs 4.0 International License (CC BY-NC-ND 4.0), which permits the non-commercial replication and distribution of the article with the strict proviso that no changes or edits are made and the original work is properly cited (including links to both the formal publication through the relevant DOI and the license). See: https://creativecommons.org/licenses/by-nc-nd/4.0/.
References
- Aletaha D, Smolen JS. Diagnosis and Management of Rheumatoid Arthritis: A Review. JAMA 2018;320:1360-72. [Crossref] [PubMed]
- McInnes IB, Schett G. Pathogenetic insights from the treatment of rheumatoid arthritis. Lancet 2017;389:2328-37. [Crossref] [PubMed]
- Zhang L, Wei W. Anti-inflammatory and immunoregulatory effects of paeoniflorin and total glucosides of paeony. Pharmacol Ther 2020;207:107452. [Crossref] [PubMed]
- Zhang L, Yu J, Wang C, et al. The effects of total glucosides of paeony (TGP) and paeoniflorin (Pae) on inflammatory-immune responses in rheumatoid arthritis (RA). Funct Plant Biol 2019;46:107-17. [Crossref] [PubMed]
- Zhang W, Dai SM. Mechanisms involved in the therapeutic effects of Paeonia lactiflora Pallas in rheumatoid arthritis. Int Immunopharmacol 2012;14:27-31. [Crossref] [PubMed]
- Liu X, Li X, Li X, et al. The efficacy and safety of total glucosides of peony in the treatment of primary Sjögren’s syndrome: a multi-center, randomized, double-blinded, placebo-controlled clinical trial. Clin Rheumatol 2019;38:657-664. Erratum in: Clin Rheumatol 2018 Dec 3. [Crossref] [PubMed]
- Zheng Q, Jiang W, Sun X, et al. Total glucosides of paeony for the treatment of psoriasis: A systematic review and meta-analysis of randomized controlled trials. Phytomedicine 2019;62:152940. [Crossref] [PubMed]
- Huang Y, Wang H, Chen Z, et al. Synergistic and Hepatoprotective Effect of Total Glucosides of Paeony on Ankylosing Spondylitis: A Systematic Review and Meta-Analysis. Front Pharmacol 2019;10:231. [Crossref] [PubMed]
- Qin Y, Tian YP. Protective effects of total glucosides of paeony and the underlying mechanisms in carbon tetrachloride-induced experimental liver injury. Arch Med Sci 2011;7:604-12. [Crossref] [PubMed]
- Chen Z, Li XP, Li ZJ, et al. Reduced hepatotoxicity by total glucosides of paeony in combination treatment with leflunomide and methotrexate for patients with active rheumatoid arthritis. Int Immunopharmacol 2013;15:474-7. [Crossref] [PubMed]
- Lin J, Xiao L, Ouyang G, et al. Total glucosides of paeony inhibits Th1/Th17 cells via decreasing dendritic cells activation in rheumatoid arthritis. Cell Immunol 2012;280:156-63. [Crossref] [PubMed]
- Li HL, Chen C, Chen C. Network pharmacology-based approach to investigate the mechanism of Huang-Lian-Jie-Du-Decoction for treatment of type 2 diabetes mellitus. Tradit Med Res 2021;6:36. [Crossref]
- Kong J, Liu CX, Zhou JL. System pharmacology-based dissection of the potential effective material basis and mechanism of Xiao-xian-xiong decoction for type 2 diabetes mellitus. Tradit Med Res 2021;6:37. [Crossref]
- Lounkine E, Keiser MJ, Whitebread S, et al. Large-scale prediction and testing of drug activity on side-effect targets. Nature 2012;486:361-7. [Crossref] [PubMed]
- Ru J, Li P, Wang J, et al. TCMSP: a database of systems pharmacology for drug discovery from herbal medicines. J Cheminform 2014;6:13. [Crossref] [PubMed]
- Keiser MJ, Roth BL, Armbruster BN, et al. Relating protein pharmacology by ligand chemistry. Nat Biotechnol 2007;25:197-206. [Crossref] [PubMed]
- Wishart DS, Knox C, Guo AC, et al. DrugBank: a knowledgebase for drugs, drug actions and drug targets. Nucleic Acids Res 2008;36:D901-6. [Crossref] [PubMed]
- Rebhan M, Chalifa-Caspi V, Prilusky J, et al. GeneCards: a novel functional genomics compendium with automated data mining and query reformulation support. Bioinformatics 1998;14:656-64. [Crossref] [PubMed]
- Piñero J, Bravo À, Queralt-Rosinach N, et al. DisGeNET: a comprehensive platform integrating information on human disease-associated genes and variants. Nucleic Acids Res 2017;45:D833-9. [Crossref] [PubMed]
- Hamosh A, Scott AF, Amberger J, et al. Online Mendelian Inheritance in Man (OMIM), a knowledgebase of human genes and genetic disorders. Nucleic Acids Res 2002;30:52-5. [Crossref] [PubMed]
- Davis AP, Grondin CJ, Johnson RJ, et al. Comparative Toxicogenomics Database (CTD): update 2021. Nucleic Acids Res 2021;49:D1138-43. [Crossref] [PubMed]
- Kanehisa M, Goto S. KEGG: kyoto encyclopedia of genes and genomes. Nucleic Acids Res 2000;28:27-30. [Crossref] [PubMed]
- Berman HM, Westbrook J, Feng Z, et al. The Protein Data Bank. Nucleic Acids Res 2000;28:235-42. [Crossref] [PubMed]
- Trott O, Olson AJ. AutoDock Vina: improving the speed and accuracy of docking with a new scoring function, efficient optimization, and multithreading. J Comput Chem 2010;31:455-61. [PubMed]
- Yuan S, Chan HCS, Filipek S, et al. PyMOL and Inkscape Bridge the Data and the Data Visualization. Structure 2016;24:2041-2. [Crossref] [PubMed]
- Wu Y, Jiang Y, Zhang L, et al. Green and efficient extraction of total glucosides from Paeonia lactiflora Pall. ‘Zhongjiang’by subcritical water extraction combined with macroporous resin enrichment. Ind Crops Prod 2019;141:111699. [Crossref]
- Liu P, Zhang Y, Gao J, et al. HPLC-DAD analysis of 15 monoterpene glycosides in oil peony seed cakes sourced from different cultivation areas in China. Ind Crops Prod 2018;118:259-70. [Crossref]
- Liu P, Xu Y, Gao X, et al. Optimization of ultrasonic-assisted extraction of oil from the seed kernels and isolation of monoterpene glycosides from the oil residue of Paeonia lactiflora Pall. Ind Crops Prod 2017;107:260-70. [Crossref]
- Jiang H, Li J, Wang L, et al. Total glucosides of paeony: A review of its phytochemistry, role in autoimmune diseases, and mechanisms of action. J Ethnopharmacol 2020;258:112913. [Crossref] [PubMed]
- Ikeda N, Fukuda T, Jyo H, et al. Quality evaluation on Paeoniae radix. I. Quantitative analysis of monoterpene glycosides constituents of Paeoniae radix by means of high performance liquid chromatography. Comparative characterization of the external figures, processing method and the cultivated areas. Yakugaku Zasshi 1996;116:138-47. [Crossref] [PubMed]
- Xiang N, Li XM, Zhang MJ, et al. Total glucosides of paeony can reduce the hepatotoxicity caused by Methotrexate and Leflunomide combination treatment of active rheumatoid arthritis. Int Immunopharmacol 2015;28:802-7. [Crossref] [PubMed]
- Cai Z, Liu J, Bian H, et al. Albiflorin alleviates ovalbumin (OVA)-induced pulmonary inflammation in asthmatic mice. Am J Transl Res 2019;11:7300-9. [PubMed]
- Zhou X, Fouda S, Zeng XY, et al. Characterization of the Therapeutic Profile of Albiflorin for the Metabolic Syndrome. Front Pharmacol 2019;10:1151. [Crossref] [PubMed]
- Ley K, Laudanna C, Cybulsky MI, et al. Getting to the site of inflammation: the leukocyte adhesion cascade updated. Nat Rev Immunol 2007;7:678-89. [Crossref] [PubMed]
- Vestweber D. How leukocytes cross the vascular endothelium. Nat Rev Immunol 2015;15:692-704. [Crossref] [PubMed]
- Baeten D, Demetter P, Cuvelier C, et al. Comparative study of the synovial histology in rheumatoid arthritis, spondyloarthropathy, and osteoarthritis: influence of disease duration and activity. Ann Rheum Dis 2000;59:945-53. [Crossref] [PubMed]
- Rao SP, Wang Z, Zuberi RI, et al. Galectin-3 functions as an adhesion molecule to support eosinophil rolling and adhesion under conditions of flow. J Immunol 2007;179:7800-7. [Crossref] [PubMed]
- Auvynet C, Moreno S, Melchy E, et al. Galectin-1 promotes human neutrophil migration. Glycobiology 2013;23:32-42. [Crossref] [PubMed]
- Hirashima M, Kashio Y, Nishi N, et al. Galectin-9 in physiological and pathological conditions. Glycoconj J 2002;19:593-600. [Crossref] [PubMed]
- Krześlak A, Lipińska A. Galectin-3 as a multifunctional protein. Cell Mol Biol Lett 2004;9:305-28. [PubMed]
- Bi S, Hong PW, Lee B, et al. Galectin-9 binding to cell surface protein disulfide isomerase regulates the redox environment to enhance T-cell migration and HIV entry. Proc Natl Acad Sci U S A 2011;108:10650-5. [Crossref] [PubMed]
- Kojima R, Ohno T, Iikura M, et al. Galectin-9 enhances cytokine secretion, but suppresses survival and degranulation, in human mast cell line. PLoS One 2014;9:e86106. [Crossref] [PubMed]
- Dai SY, Nakagawa R, Itoh A, et al. Galectin-9 induces maturation of human monocyte-derived dendritic cells. J Immunol 2005;175:2974-81. [Crossref] [PubMed]
- Sayers TJ, Mason LH, Wiltrout TA. Trafficking and activation of murine natural killer cells: differing roles for IFN-gamma and IL-2. Cell Immunol 1990;127:311-26. [Crossref] [PubMed]
- Maruotti N, Cantatore FP, Crivellato E, et al. Angiogenesis in rheumatoid arthritis. Histol Histopathol 2006;21:557-66. [PubMed]
- Lainer-Carr D, Brahn E. Angiogenesis inhibition as a therapeutic approach for inflammatory synovitis. Nat Clin Pract Rheumatol 2007;3:434-42. [Crossref] [PubMed]
- Taylor PC. VEGF and imaging of vessels in rheumatoid arthritis. Arthritis Res 2002;4:S99-107. [Crossref] [PubMed]
- Hosaka S, Shah MR, Barquin N, et al. Expression of basic fibroblast growth factor and angiogenin in arthritis. Pathobiology 1995;63:249-56. [Crossref] [PubMed]
- Cao R, Eriksson A, Kubo H, et al. Comparative evaluation of FGF-2-, VEGF-A-, and VEGF-C-induced angiogenesis, lymphangiogenesis, vascular fenestrations, and permeability. Circ Res 2004;94:664-70. [Crossref] [PubMed]
- Zhang Y, Qiu H, Zhang H, et al. Vascular endothelial growth factor A (VEGFA) polymorphisms in Chinese patients with rheumatoid arthritis. Scand J Rheumatol 2013;42:344-8. [Crossref] [PubMed]
- Burmester GR, Pope JE. Novel treatment strategies in rheumatoid arthritis. Lancet 2017;389:2338-48. [Crossref] [PubMed]
- Tamilarasan KP, Kolluru GK, Rajaram M, et al. Thalidomide attenuates nitric oxide mediated angiogenesis by blocking migration of endothelial cells. BMC Cell Biol 2006;7:17. [Crossref] [PubMed]
- D'Amato RJ, Loughnan MS, Flynn E, et al. Thalidomide is an inhibitor of angiogenesis. Proc Natl Acad Sci U S A 1994;91:4082-5. [Crossref] [PubMed]
- Jones D. PRKCa: Identification of a Novel Downstream Target of WT1. 2013. Available online: https://digitalcommons.library.tmc.edu/cgi/viewcontent.cgi?article=1411&context=utgsbs_dissertations
- Teng F, Zhang JX, Chen Y, et al. LncRNA NKX2-1-AS1 promotes tumor progression and angiogenesis via upregulation of SERPINE1 expression and activation of the VEGFR-2 signaling pathway in gastric cancer. Mol Oncol 2021;15:1234-55. [Crossref] [PubMed]
- Reeves E, James E. Tumour and placenta establishment: The importance of antigen processing and presentation. Placenta 2017;56:34-9. [Crossref] [PubMed]
- Haroon N, Inman RD. Endoplasmic reticulum aminopeptidases: Biology and pathogenic potential. Nat Rev Rheumatol 2010;6:461-7. [Crossref] [PubMed]
- Jung E, Jung W, Park SB, et al. Anti-angiogenic effect of EGHB010, a standardized herbal formula of Paeoniae radix and Glycyrrhizae radix. Pak J Pharm Sci 2020;33:129-34. [PubMed]
- Dong X, He Z, Xiang G, et al. Paeoniflorin promotes angiogenesis and tissue regeneration in a full-thickness cutaneous wound model through the PI3K/AKT pathway. J Cell Physiol 2020;235:9933-45. [Crossref] [PubMed]
- Yuan R, Shi W, Xin Q, et al. Tetramethylpyrazine and Paeoniflorin Inhibit Oxidized LDL-Induced Angiogenesis in Human Umbilical Vein Endothelial Cells via VEGF and Notch Pathways. Evid Based Complement Alternat Med 2018;2018:3082507. [Crossref] [PubMed]
- Liu R, Zheng Y, Han T, et al. Angiogenic Actions of Paeoniflorin on Endothelial Progenitor Cells and in Ischemic Stroke Rat Model. Am J Chin Med 2021;49:863-81. [Crossref] [PubMed]
- Arts EE, Fransen J, den Broeder AA, et al. The effect of disease duration and disease activity on the risk of cardiovascular disease in rheumatoid arthritis patients. Ann Rheum Dis 2015;74:998-1003. [Crossref] [PubMed]
- Wienke J, Mertens JS, Garcia S, et al. Biomarker profiles of endothelial activation and dysfunction in rare systemic autoimmune diseases: implications for cardiovascular risk. Rheumatology (Oxford) 2021;60:785-801. [Crossref] [PubMed]
- Kanda T, Takahashi T. Interleukin-6 and cardiovascular diseases. Jpn Heart J 2004;45:183-93. [Crossref] [PubMed]
- Sun J, Axelsson J, Machowska A, et al. Biomarkers of Cardiovascular Disease and Mortality Risk in Patients with Advanced CKD. Clin J Am Soc Nephrol 2016;11:1163-72. [Crossref] [PubMed]
- Mahmoudi M, Siassi F, Mahmoudi MJ, et al. Defective T-cell Proliferation and IL-2 Production in a Subgroup of Patients with Coronary Artery Disease. Iran J Allergy Asthma Immunol 2010;9:133-40. [PubMed]
- Sellke FW, Ruel M. Vascular growth factors and angiogenesis in cardiac surgery. Ann Thorac Surg 2003;75:S685-90. [Crossref] [PubMed]
- Braile M, Marcella S, Cristinziano L, et al. VEGF-A in Cardiomyocytes and Heart Diseases. Int J Mol Sci 2020;21:5294. [Crossref] [PubMed]
- Luo C, Wang H, Chen X, et al. Protection of H9c2 rat cardiomyoblasts against oxidative insults by total paeony glucosides from Radix Paeoniae Rubrae. Phytomedicine 2013;21:20-4. [Crossref] [PubMed]
- Li J, Chen CX, Shen YH. Effects of total glucosides from paeony (Paeonia lactiflora Pall) roots on experimental atherosclerosis in rats. J Ethnopharmacol 2011;135:469-75. [Crossref] [PubMed]
- Naveed M, Han L, Hasnat M, et al. Suppression of TGP on myocardial remodeling by regulating the NF-κB pathway. Biomed Pharmacother 2018;108:1460-8. [Crossref] [PubMed]