Advanced radiotherapy for metastatic disease—a major stride or a futile effort?
Introduction
Radiation Oncologists are often involved in a patient’s care from time of cancer diagnosis to end of life. It is no surprise then that 30–40% of radiation therapy treatment courses are delivered with palliative intent (1). This necessitates that practicing Radiation Oncologists be cognizant of appropriate uses of advanced therapies to maximize the quality of patient care and life. This requisite is becoming more important with the development of life-prolonging targeted therapy and immunotherapies for patients with metastatic disease.
Despite the abundance of palliative radiotherapy, many physicians report not having adequate knowledge in this competency (2). Further complicating this issue, an increasing number of physicians are encountering clinical scenarios that strike a ‘middle ground’ between purely palliative or curative intent; that is, the situation in which aggressive palliation is warranted to provide durable local control and potentially improve progression free and overall survival (3,4).
The article is intended to provide a framework that clinicians can utilize when considering treatment options in complex palliative settings. The following introductory section aims to explore the basics of advanced techniques, their relevance with respect to histology, and the importance of dosing and fractionation. This will be followed by disease site based discussions with accompanying tables summarizing relevant prospective literature utilizing advanced technologies (Tables 1-7).
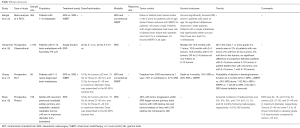
Full table
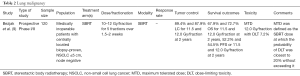
Full table
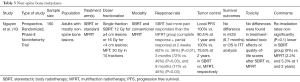
Full table
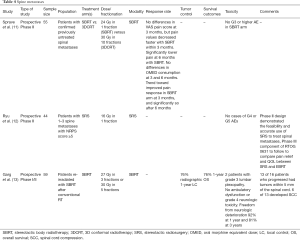
Full table
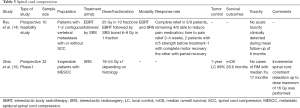
Full table
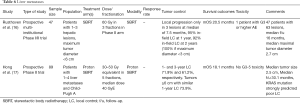
Full table
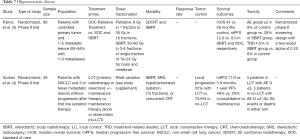
Full table
Advanced techniques
Advanced techniques wallow practitioners to escalate dose which may improve therapeutic efficacy. This is possible because such technologies permit the delivery of highly conformal treatments which maximally spare ladjacent organs at risk, theoretically reducing acute and late side effects. These improved techniques notably come at the expense of increased indirect and direct costs, thus physicians should be armed with the appropriate knowledge and tools to justify their utilization.
Intensity modulated radiotherapy (IMRT) and volumetric modulated arc therapy (VMAT) utilize inverse planning software to optimize radiation beam arrangements such that target volumes are given maximal conformal doses, while dose to organs at risk is minimized. This is in contrast to traditional forward planned 3D conformal radiotherapy in which beams are arranged at the outset, and target coverage and dose to organs at risk are analyzed after the plan has been created.
Stereotactic body radiotherapy (SBRT) involves the use of highly conformal ablative doses of radiation to tumors outside the central nervous system with a small margin of surrounding tissue. This is typically limited to a maximum of 5 fractions. SBRT allows the practitioner to deliver higher biological effective dose (BED) compared to standard fractionation courses. Stereotactic radiotherapy (SRT) is similar to SBRT in that it delivers highly conformal ablative doses of radiation in up to 5 fractions (or one fraction in stereotactic radiosurgery, SRS), however, this technique specifically targets tumors within the brain. It is also worth noting that the type of stereotactic treatment device may not be critical. Practitioners and treatment planners are likely able to accomplish similar results with different SBRT capable machines. Moreover, central to any advanced technique are the use of image guided radiotherapy (IGRT) and the expertise and experience of the treatment team. While IGRT’s role in conventional techniques may be questionable in palliative radiotherapy, practitioners should utilize image guidance with advanced techniques given the highly focal nature of therapy.
Importantly, the theoretical improvements in efficacy and reduction in side effects of the above technologies are borne out in the literature. For example, improvement in overall survival was noted in a 2004 phase III randomized trial (RTOG 9508) with the addition of SRS boost to conventional whole brain radiotherapy (WBRT) compared to WBRT alone in patients with a single brain metastasis (18). More recently, a 2015 meta-analysis also demonstrated a survival benefit with SRS alone in select patients <50 years of age with 1–4 brain metastases yover SRS plus WBRT (5) (Table 1). Better neurocognitive outcomes have also been noted with SRS alone versus SRS plus WBRT in other studies (7,19). Yamamoto et al., have also published a single arm study demonstrating the non-inferiority of SRS without WBRT in patients with five to ten metastases compared with those with two to four lesions (6) (Table 1).
Nevertheless, as patients may not live long enough to reap the benefit of advanced technologies, the use of such techniques remains an explorable topic. This is especially pertinent as the pace at which IMRT and SRS are being utilized in clinical practice is faster than the rate of evidence supporting their use in both in the metastatic setting and in the last 30 days of life (20,21).
Histology
It is important to note that certain tumor types are more ‘radiosensitive’ than others. Classic examples of the former include hematologic malignancies, seminoma, breast carcinoma, prostate adenocarcinoma, and ovarian carcinoma. Examples of ‘radioresistant’ malignancies include renal cell carcinoma (RCC), melanoma, sarcoma, thyroid cancer, hepatocellular carcinoma, colorectal carcinoma, and non-small cell lung cancer (NSCLC). Radioresistant tumors require techniques delivering higher BEDs (i.e., SBRT, SRS, or SRT) to achieve similar rates of local control compared to their radiosensitive counterparts. A study from the Mayo Clinic demonstrated that patients with metastatic melanoma to the brain who received a BED >39.0 Gray (Gy) were found to have longer periods of palliation and freedom from progression than those receiving less dose (22).
Dose and fractionation
A nuanced area in palliative care resides in decision regarding dose and fractionation. This decision depends on a host of factors including performance status, estimated prognosis, prior lines of treatment, current comorbidities, acute toxicities, and whether the patient is currently under active systemic therapy (23). The selection of dose must also be carefully chosen in those with limited life expectancies, 1 with particular emphasis on delivering effective regimens with the fewest number of fractions (i.e., limiting the time the patient is on treatment). Importantly, many meta-analyses show similar outcomes for shorter and longer courses of radiotherapy with respect to palliative endpoints (24). In patients receiving palliative radiotherapy with the intent to provide durable control, as highlighted above, higher BED dose and fractionation is likely warranted given the increased control rates associated with higher doses. If SBRT is offered for palliation of symptoms, in the determination of dose and fractionation, one will have to consider the therapeutic ratio (benefits vs. risk) as it is imperative that the treatment should not cause significant toxicities which will offset the benefits of the treatment.
Further, while the effects of palliative radiotherapy are not limited by histology or anatomical subsite, some tumor histologies are able to repair themselves more efficiently which should be taken into account (25).
Prognostication
Unfortunately, estimation of prognosis has remained a challenging area for clinicians, especially with respect to palliative oncology (26). In one study, time remaining was overestimated by a factor of 5.3 (27). To help address this known deficiency, clinicians can utilize a number of different prognostication tools. These tools are reviewed in more detail elsewhere in this issue.
Importantly, clinicians should critically think about whether radiotherapy will actually improve the length of survival. If the answer is likely not, then the use of advanced technologies may only be warranted if the intent is providing durable local control in high risk disease or to minimize side effects or complications as in reirradiation of spinal metastasis. There is unfortunately a lack of data on the impact of advanced techniques on side effects for patients receiving palliative radiotherapy. As such, the consideration of whether advanced techniques will minimize side effect profile should be made on a case-by-case basis when evaluating treatment plans. If a more traditional therapy, such as 3D conformal therapy, is likely to be similar in terms of side effects a more advanced technique may not be necessary.
Brain metastases
Brain metastases are more common with certain histologies and have the potential to cause significant morbidity and mortality given the fixed volume of the cranium and eloquent substructures that reside within it. With this diagnosis, is important to be thoughtful about the modality of treatment (i.e., surgery vs. SRS vs. WBRT) as survival spans from 2.8 to 25.3 months depending on prognostic variables. The use of the Diagnosis-Specific Graded Prognostic Assessment (DS-GPA) is helpful in predicting life expectancy and can help tailor management based on a multitude of factors (28). The most prognostically favorable patients tend to be younger (<50 years old) with KPS 90-100, 1 intracranial metastasis and have no extracranial metastases (29).
Median survivals for NSCLC, small cell lung cancer, melanoma, RCC, breast cancer, and GI cancers are 7.00, 4.90, 6.74, 9.63, 13.80, and 5.36 months based on DS-GPA (published in 2012), respectively (28). While this data is robust, it is also important to note that patients with brain metastases are increasingly surviving for longer periods with improved systemic therapies. This is especially evident when comparing survival rates from the RPA (a 1997 model) where the best performing patients had a median survival of 7.1 months, followed by 4.2 and 2.3 months for class II and III patients.
When considering the role of radiotherapy, it is important to note that the use of WBRT in combination with surgery or SRS does not confer an overall survival benefit and can cause deleterious effects on quality of life (7) (Table 1). With respect to dose and fractionation with WBRT, there is no difference in overall survival or symptom control between 30 Gy in 10 fractions or 20 Gy in 5 fractions. The use of a shorter course may be the most reasonable in patients with shorter life expectancies.
Advanced technologies, such as SRS, have demonstrated a high local control benefit in patients with oligometastatic disease. Advantages of this therapy include its highly focal minimally invasive approach, and that it can be delivered synergistically with immunotherapy. There is additional prospective evidence that it can be delivered in patients with up to 10 brain metastases (30). For patients with limited numbers of brain metastases, SRS offers the ability to deliver highly conformal treatment without the neurocognitive side effects of WBRT. It should be noted that the highly focal nature of therapy comes with the risk of distant brain metastases and no change in overall survival (31).
A not infrequent and complex situation in palliative brain radiotherapy is that of re-irradiation. Previous publications have demonstrated the efficacy and safety of retreatment. Mariya et al. reported that repeat SRS is an effective treatment option, leading to a long survival with a decreased neurological decline. The authors analyzed 28 patients who underwent salvage radiosurgery for recurrent brain metastases from NSCLC showing a median survival from initial SRS of 26 and 11 months for repeat SRS (32,33). RTOG 90-05 also demonstrated the feasibility of retreatment with SRS of a recurrent primary tumor and metastatic brain tumors that were previously irradiated. The authors further demonstrated that the maximally tolerated doses ranged between 15 and 24 Gy (8) (Table 1). With regard to neurological complications of repeat SRS, Bhatnagar et al. reported that treatment was performed with minimal CNS toxicity compared with the baseline in 26 patients with benign and malignant tumors (34).
There is a range of data regarding outcomes with re-irradiation. In addition to the above mentioned studies Kwon et al. reported a median survival time of 8 months from the time of repeat SRS for recurrence/progressive disease in 43 patients (35). Another study reported median survival times in patients who repeated SRS to recurrent or newly developed metastases of 15 and 22.4 months, respectively (36,37). Chen et al. also analyzed retreatment with salvage radiosurgery and demonstrated a median survival time of SRS for recurrent brain metastases of 7 months in 45 patients (38).
Importantly, in the case of re-irradiation with SRS preceded by SRS, there is still insufficient evidence to generate definitive treatment recommendations, and the decision must be made on an individualized basis (39).
One alternative advanced technique is that of hippocampal sparing brain IMRT. This therapy is a seeming “middle ground” between SRS and WBRT as it prevents distant brain metastases while sparing the patient of neurocognitive side effects that tend to occur with traditional WBRT (40). Physicians should otherwise also factor in the increased resource utilizations rates for advanced technologies especially when delivering highly conformal treatments with daily IGRT.
Lung malignancy
When contemplating palliative treatment modalities for this disease site, physicians should think critically about the role of minimally invasive stereotactic body radiotherapy (SBRT). This is especially so as SBRT has the potential to improve overall survival for patients with oligometastatic lung lesions, albeit with a 20% increase in grade 2 or higher toxicity (3).
Further, given the ablative nature of SBRT and high associated primary tumor control rates, SBRT has the ability to palliate aggressive lesions which have the potential to compromise respiratory function or grow more centrally into the mediastinum. Moreover, while SBRT was traditionally avoided in centrally located lesions given the higher risk of toxicity, recent prospective data has demonstrated low rates of dose limiting toxicity with up to 12.0 Gy/fraction SBRT for tumors <5 centimeters with high rates of control (>87% at 2 years) (9) (Table 2). While this trial included only patients with primary lung malignancy, it lends support to considering the delivery of ablative dose in traditionally avoided locations.
Ultimately, if a patient’s disease burden is not overwhelming, SBRT can provide meaningful palliation and should be considered for therapy. While no trial of SBRT in lung metastases has demonstrated symptom improvement, it remains a viable hypothesis that enhancing tumor control with higher BED regimens can translate to improved symptom control if tumor progression is contained in critical areas. The abbreviated nature of therapy also has benefit of improved patient quality of life and travel burden.
One other notable option at certain treatment centers is proton therapy. This unique modality has the benefit of minimizing exit dose and sparing local surrounding tissue based on the physical properties of the particle. This treatment can be considered in cases of re-irradiation where there is particular benefit in avoiding previously treated areas or structures.
Non-spine bone metastases
Convention external beam radiation therapy (EBRT) offers well tolerated therapy when combined with other palliative agents for bone metastases (41). Rates of complete overall pain relief on an intention-to-treat analysis in a recently published meta-analysis were similar in patients for single fraction treatments and multiple fraction treatments (61% and 62%, respectively). Complete response rates were very similar as well (23% and 24%, respectively) (42). Notably, a variety of different dose and fractionation schemes for uncomplicated metastases have shown equivalent pain relief in randomized controlled trials (42). Single fraction radiotherapy does not produce unacceptable rates of long-term effects and has been found to be underutilized in clinical practice (43). Effective palliation with retreatment has also been shown to be safe on updated data analyses (24).
Importantly, SBRT has been shown to yield high rates of long-term local control for non-spine bone metastases with a low fracture risk (44). Moreover, a recently published phase II trial by Nguyen et al. demonstrated higher rates of pain response (both complete and partial) compared to multifraction radiotherapy (MFRT) (10) (Table 3). This analysis of 160 patients with confirmed painful bone metastases randomized patients to receive single fraction SBRT (12 or 16 Gy based on tumor size) or MFRT (30 Gy in 10 fractions). Among patients who were evaluable, the SBRT group had higher pain response at 2 weeks, 3, and 9 months without differences in toxicities or quality of life scores. Further bolstering the utility of SBRT is that local control rates were improved in the SBRT arm (100% vs. 90.5% and 100% vs. 75.6% at 1 and 2 years, respectively).
Critical judgment is thus required by the physician in deciding dosing and fractionation and whether to utilize advanced techniques such as SBRT. SBRT may be most appropriate in those patients with good performance status, reasonably long life expectancy, a tumor histology that is more likely respond to higher BEDs, and locations in which durable local control may be of significant importance. Notably, medical agents such as bisphosphonates can also be considered as alternatives to radiotherapy in the treatment paradigm.
Spine metastases and spinal cord compression
Spine metastases present a unique situation in palliative radiotherapy. Classic palliative radiation is typically delivered with the goal of providing rapid and durable symptom relief, minimizing side effects and minimizing patient and family burden. However, in this circumstance an important additional goal of radiotherapy is providing durable local control so as to prevent fracture or spinal cord compression. SBRT in particular delivers significantly higher BED, more precisely, and in a shorter time frame. However, the treatment goal (i.e., ablation) is different than the goals of traditional palliative radiation therapy.
Given this, direct comparisons between SBRT and conventional palliative radiotherapy is challenging as the endpoints are not usually matched. Interestingly, however, a recent randomized phase II trial from the University of Heidelberg demonstrated that SBRT may confer an advantage over conventionally fractionated radiotherapy with respect to pain control (11) (Table 4). Moreover, a phase II study (RTOG 0631) comparing SBRT with single fraction EBRT demonstrated promising results with respect to feasibility and accurate use of SBRT to treat spinal metastases (12) (Table 4). We are eagerly awaiting the results of the phase III component of the same study.
An important consideration when deciding on the utilization of advanced technologies such as SBRT is the impact of radiation on the structural stability of the vertebral body, which has a not insignificant risk of therapy related vertebral fracture (14% in one study) (45). To help decide regarding treatment techniques (i.e., conventional fractionation vs. SBRT vs. surgery) physicians can use validated criteria such as the Spinal Instability Neoplastic Score (SINS) which evaluates spinal stability (46).
Given the proximity of spine metastases to critical structures such as the spinal cord, clinicians should also consider degrees of epidural extension evaluated by the Bilsky score (47). Grade II and III disease may warrant traditional fractionation over SBRT if surgical decompression is not considered as the proximity of the tumor to the spinal cord may not be amenable to high dose per fraction therapy in spite of the rapid dose fall off. Patients with grade I disease on the other hand may be better candidates for SBRT.
Advanced technologies can otherwise offer advantages in patients who have had prior RT where normal tissue tolerance is at its limit, especially with respect to the spinal cord (i.e., preventing radiation induced myelopathy) (48,49). SBRT can also be useful in the avoidance of other critical organs such as the bowel.
Taken together, we believe optimal inclusion criteria for spine SBRT are patients with good to excellent performance status, have oligometastatic disease, have no more than 3 spinal levels involved, have no or minimal spinal instability or high grade epidural disease, have a radioresistant tumor histology, and have not had any prior conventional EBRT to the affected level (or at least 5 months from delivery of prior therapy).
Importantly, given the increased cost associated with advanced techniques (conventional EBRT is approximately 29–71% of the cost of SBRT) clinicians should attempt to estimate the prognosis of patients and consider whether the patient will live long enough to deem the treatment cost effective (50,51). Potential prognostic models for patients with spinal metastases include the Revised Tokuhashi score [2005], Tomita score [2001], and Modified Baur score.
Regarding the setting of re-irradiation, Chow et al. published data that suggested that patients requiring repeat radiation therapy could be reasonably retreated with conventional 8 Gy in 1 fraction (52). Other studies have also demonstrated no difference between single fraction radiotherapy and multifraction therapy, except in patients with SINS scores >11 with single fraction therapy (53,54). With respect to SBRT re-irradiation, Garg et al. have published results evaluating 27–30 Gy in 3–5 fractions after conventional palliative radiotherapy. One year radiographic local control and overall survival in 59 patients were both 76% with acceptable toxicity, most commonly grade 1 or 2 fatigue. Two patients experienced mild to moderate lumbar plexopathy without ambulatory dysfunction (13) (Table 4). Mahadevan et al. also reported their outcomes of SBRT re-irradiation for recurrent epidural spinal metastases. Sixty patients were treated to 24–30 Gy in 3–5 fractions depending on tumor proximity to the spinal cord. Median overall survival was 11 months and median progression free survival was 8 months without any significant toxicity aside from fatigue. Ninety-three percent of patients had stable or improved disease and 65% experienced pain relief (55).
Spratt et al. (56) have developed an integrated multidisciplinary algorithm for spinal metastases which can be used as a guide.
Spinal cord compression (SCC)
SCC is a unique situation in which timely radiotherapy must be delivered with or without neurosurgery to prevent long term deficits. Treatment decisions in this scenario must be informed by the patient’s overall clinical trajectory, prognosis, histology, symptoms, and patient preferences.
Patchell et al., published data suggesting that patients with SCC had superior outcomes in the end points of ability to walk and retention of ability to walk with combination surgery and radiotherapy compared to radiotherapy alone (57). As such, consultation with Neurosurgery should always be considered in this clinical scenario.
When considering prescription dose, longer dose and fractionation schemes were found to have higher local control in one trial (58). Further, higher BED techniques (such as SBRT) are more likely to control tumors compressing the cord. The latter aspect is a more important consideration in patients with longer life expectancies.
Currently, we have data to support the feasibility of stereotactic radiotherapy for SCC and colleagues from Henry Ford Hospital and MD Anderson Cancer Center have reported their experience with the use of single fraction SBRT for epidural spinal cord compression with promising results (14,15,59) (Table 5). However, SBRT is a very labor-intensive procedure and even with a generalizable class solution, it can take a few days for the planning and quality assurance process to be completed and neurological deterioration can occur during that time (60). As such, the potential benefits of SBRT should be weighed against the urgency of the clinical scenario, especially when considering the significantly reduced planning time associated with 2D or 3D conformal therapy.
Moreover, if a patient has a relative short life expectancy (<3 months), we would encourage clinicians to strongly consider a short course of radiotherapy as there is no difference in motor function or overall survival. Recent data suggests that short course radiotherapy is as effective as long course therapy in patients with poor prognosis (61). To estimate prognosis, clinicians can utilize any one of the number of validated scoring criteria that are discussed elsewhere in this issue.
Liver metastases
Single fraction liver radiotherapy (8 Gy in 1 fraction) has been demonstrated previously to be useful in the palliation of a substantial proportion (approximately 48%) of patients with pain or abdominal discomfort from liver metastases with minor toxicity (62). However, SBRT has become an accepted form of therapy in patients with oligometastatic disease if treated lesions can be kept to normal tissue constraints (63).
One study demonstrated 90–100% local control at 2 years after treatment of patients with 1–3 liver metastases with dose escalated SBRT (36 to 60 Gy in 3 fractions) with a median survival of 20.5 months (16) (Table 6).
At certain institutions, conventionally fractionated or hypofractionated proton beam therapy is a potential option for patients. The advantage of this therapy is the significant dose fall off beyond the tumor, allowing more normal tissue to be potentially spared of acute or late radiation effects. Protons are particularly useful in the case of larger volume metastases where it is critically important to spare remaining healthy liver parenchyma or adjacent at risk organs.
Notably, therapy can also be advanced as proton SBRT. A phase II study of proton-based SBRT for liver metastases demonstrated no grade 3 to 5 toxicity and 1- and 3-year local control rates of 71.9% and 61.2%, respectively. Protons were effective even for tumors that were 6 centimeters or larger (17) (Table 6).
Importantly, optimal patient selection is required with high dose photon or proton therapy as patients have the potential to suffer from hepatic decompensation on treatment or shortly thereafter. Radiation therapy should thus typically be limited to suitable patients, such as Child-Pugh A and B patients with limited other comorbidities. Treatment plans should also meet all or most dose constraints. Treatment with proton beam therapy should primarily be considered at experienced centers due to its unique physical properties and nuanced dosimetric considerations.
Conclusions
Palliative care plays a significant role in the modern Radiation Oncology practice and the role of advanced techniques is emerging in the setting of brain metastases, lung metastases, bone metastases, spine metastases, malignant spinal cord compression, and liver metastases. Numerous trials have demonstrated the efficacy of advanced techniques in a host of disease sites and the incorporation of these techniques in standard practice should be considered.
The most important aspect for clinicians involves the process of patient selection and determining which individuals are most likely to benefit from advanced therapies. Clinicians should also incorporate global factors when deciding technique, dose and fractionation. This may include considering how quickly the patient needs to return to their systemic therapy or if he or she can tolerate extended set-up procedures for SBRT or IMRT. Importantly, this review has purposefully only briefly discussed the role of advanced therapies in oligometastatic disease, but there has notably been recent promising data supporting the use of advanced technologies in this setting as well (4,47,64) (Table 7).
While this article serves to provide a framework for clinicians in thinking about complex palliative settings, there still remain outstanding questions that need to be addressed in future studies:
- Is there a role for advanced technologies in patients with shorter prognoses?
- Is there a potential benefit to delivering single fraction SBRT over single fraction conventional radiation for non-spine bone metastases?
- Even if advanced technologies enhance local control, improve survival or reduce symptoms, are these techniques cost-effective and justifiable from a societal standpoint?
- Are there methods or processes which can be employed to decrease the cost or resource burden of advanced techniques?
Acknowledgments
None.
Footnote
Conflicts of Interest: SS Lo is a member of the ICON Gamma Knife Expert Group, Elekta AB. The other authors have no conflicts of interest to declare.
Ethical Statement: The authors are accountable for all aspects of the work in ensuring that questions related to the accuracy or integrity of any part of the work are appropriately investigated and resolved.
References
- Ferrell BR, Temel JS, Temin S, et al. Integration of Palliative Care Into Standard Oncology Care: American Society of Clinical Oncology Clinical Practice Guideline Update. J Clin Oncol 2017;35:96-112. [Crossref] [PubMed]
- Wei RL, Mattes MD, Yu J, et al. Attitudes of radiation oncologists toward palliative and supportive care in the United States: Report on national membership survey by the American Society for Radiation Oncology (ASTRO). Pract Radiat Oncol 2017;7:113-9. [Crossref] [PubMed]
- Palma DA, Olson R, Harrow S, et al. Stereotactic ablative radiotherapy versus standard of care palliative treatment in patients with oligometastatic cancers (SABR-COMET): a randomised, phase 2, open-label trial. Lancet 2019;393:2051-8. [Crossref] [PubMed]
- Gomez DR, Blumenschein GR, Lee JJ, et al. Local consolidative therapy versus maintenance therapy or observation for patients with oligometastatic non-small-cell lung cancer without progression after first-line systemic therapy: a multicenter, randomized, controlled, phase 2 study. Lancet Oncol 2016;17:1672-82. [Crossref] [PubMed]
- Sahgal A, Aoyama H, Kocher M, et al. Phase 3 trials of stereotactic radiosurgery with or without whole-brain radiation therapy for 1 to 4 brain metastases: individual patient data meta-analysis. Int J Radiat Oncol Biol Phys 2015;91:710-7. [Crossref] [PubMed]
- Yamamoto M, Serizawa T, Shuto T, et al. Stereotactic radiosurgery for patients with multiple brain metastases (JLGK0901): a multi-institutional prospective observational study. Lancet Oncol 2014;15:387-95. [Crossref] [PubMed]
- Chang EL, Wefel JS, Hess KR, et al. Neurocognition in patients with brain metastases treated with radiosurgery or radiosurgery plus whole-brain irradiation: a randomised controlled trial. Lancet Oncol 2009;10:1037-44. [Crossref] [PubMed]
- Shaw E, Scott C, Souhami L, et al. Single dose radiosurgical treatment of recurrent previously irradiated primary brain tumors and brain metastases: final report of RTOG protocol 90–05. Int J Radiat Oncol Biol Phys 2000;47:291-8. [Crossref] [PubMed]
- Bezjak A, Paulus R, Gaspar LE, et al. Safety and Efficacy of a Five-Fraction Stereotactic Body Radiotherapy Schedule for Centrally Located Non-Small-Cell Lung Cancer: NRG Oncology/RTOG 0813 Trial. J Clin Oncol 2019;37:1316-25. [Crossref] [PubMed]
- Nguyen QN, Chun SG, Chow E, et al. Single-Fraction Stereotactic vs Conventional Multifraction Radiotherapy for Pain Relief in Patients With Predominantly Nonspine Bone Metastases: A Randomized Phase 2 Trial. JAMA Oncol 2019. [Epub ahead of print]. [Crossref] [PubMed]
- Sprave T, Verma V, Förster R, et al. Randomized phase II trial evaluating pain response in patients with spinal metastases following stereotactic body radiotherapy versus three-dimensional conformal radiotherapy. Radiother Oncol 2018;128:274-82. [Crossref] [PubMed]
- Ryu S, Pugh SL, Gerszten PC, et al. RTOG 0631 phase 2/3 study of image guided stereotactic radiosurgery for localized (1-3) spine metastases: phase 2 results. Pract Radiat Oncol 2014;4:76-81. [Crossref] [PubMed]
- Garg AK, Wang X, Shiu AS, et al. Prospective evaluation of spinal reirradiation by using stereotactic body radiation therapy: The University of Texas MD Anderson Cancer Center experience. Cancer 2011;117:3509-16. [Crossref] [PubMed]
- Ryu S, Fang Yin F, Rock J, et al. Image-guided and intensity-modulated radiosurgery for patients with spinal metastasis. Cancer 2003;97:2013-8. [Crossref] [PubMed]
- Ghia AJ, Guha-Thakurta N, Hess K, et al. Phase 1 Study of Spinal Cord Constraint Relaxation With Single Session Spine Stereotactic Radiosurgery in the Primary Management of Patients With Inoperable, Previously Unirradiated Metastatic Epidural Spinal Cord Compression. Int J Radiat Oncol Biol Phys 2018;102:1481-8. [Crossref] [PubMed]
- Rusthoven KE, Kavanagh BD, Cardenes H, et al. Multi-institutional phase I/II trial of stereotactic body radiation therapy for liver metastases. J Clin Oncol 2009;27:1572-8. [Crossref] [PubMed]
- Hong TS, Wo JY, Borger DR, et al. Phase II Study of Proton-Based Stereotactic Body Radiation Therapy for Liver Metastases: Importance of Tumor Genotype. J Natl Cancer Inst 2017;109. [Crossref] [PubMed]
- Andrews DW, Scott CB, Sperduto PW, et al. Whole brain radiation therapy with or without stereotactic radiosurgery boost for patients with one to three brain metastases: phase III results of the RTOG 9508 randomised trial. Lancet 2004;363:1665-72. [Crossref] [PubMed]
- Brown PD, Jaeckle K, Ballman KV, et al. Effect of Radiosurgery Alone vs Radiosurgery With Whole Brain Radiation Therapy on Cognitive Function in Patients With 1 to 3 Brain Metastases A Randomized Clinical Trial. JAMA 2016;316:401-9. [Crossref] [PubMed]
- Guadagnolo BA, Huo J, Liao K-P, et al. Changing trends in radiation therapy technologies in the last year of life for patients diagnosed with metastatic cancer in the United States. Cancer 2013;119:1089-97. [Crossref] [PubMed]
- Guadagnolo BA, Liao KP, Giordano SH, et al. Increasing use of advanced radiation therapy technologies in the last 30 days of life among patients dying as a result of cancer in the United States. J Oncol Pract 2014;10:e269-76. [Crossref] [PubMed]
- Olivier KR, Schild SE, Morris CG, et al. A higher radiotherapy dose is associated with more durable palliation and longer survival in patients with metastatic melanoma. Cancer 2007;110:1791-5. [Crossref] [PubMed]
- Bruera E, Kuehn N, Miller MJ, et al. The Edmonton Symptom Assessment System (ESAS): a simple method for the assessment of palliative care patients. J Palliat Care 1991;7:6-9. [Crossref] [PubMed]
- Lutz S, Balboni T, Jones J, et al. Palliative radiation therapy for bone metastases: Update of an ASTRO Evidence-Based Guideline. Pract Radiat Oncol 2017;7:4-12. [Crossref] [PubMed]
- Lutz S, Korytko T, Nguyen J, et al. Palliative radiotherapy: when is it worth it and when is it not? Cancer J 2010;16:473-82. [Crossref] [PubMed]
- Gripp S, Mjartan S, Boelke E, et al. Palliative radiotherapy tailored to life expectancy in end-stage cancer patients: reality or myth? Cancer 2010;116:3251-6. [Crossref] [PubMed]
- Christakis NA, Lamont EB. Extent and determinants of error in doctors' prognoses in terminally ill patients: prospective cohort study. BMJ 2000;320:469-72. [Crossref] [PubMed]
- Sperduto PW, Kased N, Roberge D, et al. Summary report on the graded prognostic assessment: an accurate and facile diagnosis-specific tool to estimate survival for patients with brain metastases. J Clin Oncol 2012;30:419-25. [Crossref] [PubMed]
- Sperduto CM, Watanabe Y, Mullan J, et al. A validation study of a new prognostic index for patients with a brain metastases: the Graded Prognostic Assessment. J Neurosurg 2008;109:87-9. [Crossref] [PubMed]
- Wolf A, Kondziolka D. Brain metastases: radiosurgery. Handb Clin Neurol 2018;149:129-35. [Crossref] [PubMed]
- Mehta MP, Tsao MN, Whelan TJ, et al. The American Society for Therapeutic Radiology and Oncology (ASTRO) evidence-based review of the role of radiosurgery for brain metastases. Int J Radiat Oncol Biol Phys 2005;63:37-46. [Crossref] [PubMed]
- Mariya Y, Sekizawa G, Matsuoka Y, et al. Repeat stereotactic radiosurgery in the management of brain metastases from non-small cell lung cancer. Tohoku J Exp Med 2011;223:125-31. [Crossref] [PubMed]
- Mariya Y, Sekizawa G, Matsuoka Y, et al. Outcome of stereotactic radiosurgery for patients with non-small cell lung cancer metastatic to the brain. J Radiat Res 2010;51:333-42. [Crossref] [PubMed]
- Bhatnagar A, Heron DE, Kondziolka D, et al. Analysis of repeat stereotactic radiosurgery for progressive primary and metastatic CNS tumors. Int J Radiat Oncol Biol Phys 2002;53:527-32. [Crossref] [PubMed]
- Kwon KY, Kong DS, Lee JI, et al. Outcome of repeated radiosurgery for recurrent metastatic brain tumors. Clin Neurol Neurosurg 2007;109:132-7. [Crossref] [PubMed]
- Yamanaka K, Iwai Y, Yasui T, et al. Gamma Knife radiosurgery for metastatic brain tumor: the usefulness of repeated Gamma Knife radiosurgery for recurrent cases. Stereotact Funct Neurosurg 1999;72:73-80. [Crossref] [PubMed]
- Shuto T, Fujino H, Inomori S, et al. Repeated Gamma Knife radiosurgery for multiple metastatic brain tumors. Acta Neurochir (Wien) 2004;146:989-93. [Crossref] [PubMed]
- Chen JC, Petrovich Z, Giannotta SL, et al. Radiosurgical salvage therapy for patients presenting with recurrence of metastatic disease to the brain. Neurosurgery 2000;46:860-6. [PubMed]
- Ammirati M, Cobbs CS, Linskey ME, et al. The role of retreatment in the management of recurrent/progressive brain metastases: a systemic review and evidence-based clinical practice guideline. J Neurooncol 2010;96:85-96. [Crossref] [PubMed]
- Oskan F, Ganswindt U, Schwarz SB, et al. Hippocampus sparing in whole-brain radiotherapy. A review. Strahlenther Onkol 2014;190:337-41. [Crossref] [PubMed]
- Lutz S, Berk L, Chang E, et al. Palliative radiotherapy for bone metastases: an ASTRO evidence-based guideline. Int J Radiat Oncol Biol Phys 2011;79:965-76. [Crossref] [PubMed]
- Rich SE, Chow R, Raman S, et al. Update of the systematic review of palliative radiation therapy fractionation for bone metastases. Radiother Oncol 2018;126:547-57. [Crossref] [PubMed]
- Bekelman JE, Epstein AJ, Emanuel EJ. Single- vs Multiple-Fraction Radiotherapy for Bone Metastases From Prostate Cancer. JAMA 2013;310:1501-2. [Crossref] [PubMed]
- Erler D, Brotherston D, Sahgal A, et al. Local control and fracture risk following stereotactic body radiation therapy for non-spine bone metastases. Radiother Oncol 2018;127:304-9. [Crossref] [PubMed]
- Sahgal A, Atenafu EG, Chao S, et al. Vertebral compression fracture after spine stereotactic body radiotherapy: a multi-institutional analysis with a focus on radiation dose and the spinal instability neoplastic score. J Clin Oncol 2013;31:3426-31. [Crossref] [PubMed]
- Fourney DR, Frangou EM, Ryken TC, et al. Spinal instability neoplastic score: an analysis of reliability and validity from the spine oncology study group. J Clin Oncol 2011;29:3072-7. [Crossref] [PubMed]
- Bilsky MH, Laufer I, Fourney DR, et al. Reliability analysis of the epidural spinal cord compression scale. J Neurosurg Spine 2010;13:324-8. [Crossref] [PubMed]
- Sahgal A, Weinberg V, Ma L, et al. Probabilities of radiation myelopathy specific to stereotactic body radiation therapy to guide safe practice. Int J Radiat Oncol Biol Phys 2013;85:341-7. [Crossref] [PubMed]
- Lo SS, Sahgal A, Chang EL, et al. Serious complications associated with stereotactic ablative radiotherapy and strategies to mitigate the risk. Clin Oncol (R Coll Radiol) 2013;25:378-87. [Crossref] [PubMed]
- Haley ML, Gerszten PC, Heron DE, et al. Efficacy and cost-effectiveness analysis of external beam and stereotactic body radiation therapy in the treatment of spine metastases: a matched-pair analysis. J Neurosurg Spine 2011;14:537-42. [Crossref] [PubMed]
- Kim H, Rajagopalan MS, Beriwal S, et al. Cost-effectiveness analysis of single fraction of stereotactic body radiation therapy compared with single fraction of external beam radiation therapy for palliation of vertebral bone metastases. Int J Radiat Oncol Biol Phys 2015;91:556-63. [Crossref] [PubMed]
- Chow E, van der Linden YM, Roos D, et al. Single versus multiple fractions of repeat radiation for painful bone metastases: a randomised, controlled, non-inferiority trial. Lancet Oncol 2014;15:164-71. [Crossref] [PubMed]
- Howell DD, James JL, Hartsell WF, et al. Single-fraction radiotherapy versus multifraction radiotherapy for palliation of painful vertebral bone metastases-equivalent efficacy, less toxicity, more convenient: a subset analysis of Radiation Therapy Oncology Group trial 97-14. Cancer 2013;119:888-96. [Crossref] [PubMed]
- Lam TC, Uno H, Krishnan M, et al. Adverse Outcomes After Palliative Radiation Therapy for Uncomplicated Spine Metastases: Role of Spinal Instability and Single-Fraction Radiation Therapy. Int J Radiat Oncol Biol Phys 2015;93:373-81. [Crossref] [PubMed]
- Mahadevan A, Floyd S, Wong E, et al. Stereotactic Body Radiotherapy Reirradiation for Recurrent Epidural Spinal Metastases. Int J Radiat Oncol Biol Phys 2011;81:1500-5. [Crossref] [PubMed]
- Spratt DE, Beeler WH, de Moraes FY, et al. An integrated multidisciplinary algorithm for the management of spinal metastases: an International Spine Oncology Consortium report. Lancet Oncol 2017;18:e720-30. [Crossref] [PubMed]
- Patchell RA, Tibba PA, Regine WF, et al. Direct decompressive surgical resection in the treatment of spinal cord compression caused by metastatic cancer: a randomised trial. Lancet 2005;366:643-8. [Crossref] [PubMed]
- Rades D, Lange M, Veninga T, et al. Final results of a prospective study comparing the local control of short-course and long-course radiotherapy for metastatic spinal cord compression. Int J Radiat Oncol Biol Phys 2011;79:524-30. [Crossref] [PubMed]
- Lee I, Omodon M, Rock J, et al. Stereotactic radiosurgery for high-grade metastatic epidural cord compression. J Radiosurg SBRT 2014;3:51-8. [PubMed]
- Weksberg DC, Palmer MB, Vu KN, et al. Generalizable class solutions for treatment planning of spinal stereotactic body radiation therapy. Int J Radiat Oncol Biol Phys 2012;84:847-53. [Crossref] [PubMed]
- Hoskin P, Misra V, Hopkins K, et al. SCORAD III: Randomized noninferiority phase III trial of single-dose radiotherapy (RT) compared to multifraction RT in patients (pts) with metastatic spinal canal compression (SCC). J Clin Oncol 2017. [Crossref]
- Soliman H, Ringash J, Jiang H, et al. Phase II trial of palliative radiotherapy for hepatocellular carcinoma and liver metastases. J Clin Oncol 2013;31:3980-6. [Crossref] [PubMed]
- Sharma S, Hertan L, Jones J. Palliative radiotherapy: current status and future directions. Semin Oncol 2014;41:751-63. [Crossref] [PubMed]
- Salama JK, Milano MT. Radical Irradiation of Extracranial Oligometastases. J Clin Oncol. 2014;32. [Crossref] [PubMed]