Little patients, big impacts: a narrative review of palliative and emergent radiotherapy for pediatric cancers
Introduction
The primary principle of palliative radiotherapy (RT) is that radiation should improve the overall quality of life of children whose life span is shortened by their disease, where less invasive methods of symptom relief are not sufficient. However, for the pediatric patient population, there remains an information gap and underutilization of RT in the palliative setting.
RT practice patterns in a multi-institutional series demonstrated only a minority (11%) of pediatric cancer patients were treated with a palliative intent. Importantly, the range of utilization of palliative RT across different institutions was 1–28% suggestive of significant institutional variability. The physicians surveyed conveyed concerns that toxicity and logistics (travel, time away from home, financial, etc.) were frequently and/or sometimes a barrier for receiving palliative RT treatment (1). In short, for palliative RT to be successful, the benefits and risks have to be broadly defined to be patient-centered, rather than narrowly-focused on tumor symptom relief.
We seek to provide a comprehensive resource for providers to illuminate the evidence supporting RT for pediatric cancers in the palliative and emergent setting with a goal to break down the barriers for use, including how to communicate with patients and their families about this potentially healing tool. We present this article in accordance with the Narrative Review reporting checklist (available at https://apm.amegroups.com/article/view/10.21037/apm-23-505/rc).
Methods
Table 1 summarizes the search strategy performed to identify relevant articles on the use of RT in the palliative and emergent setting for pediatric patients [age ≤21 years old (yo)]. Articles were analyzed for relevance, histology specific information including dose and fractionation, and quality of data. There were no prospective studies identified on the use of RT in the palliative setting for pediatric patients. These articles were supplemented with additional current and recent clinical trials, review papers, and survey studies. In areas that lacked pediatric specific data, well-designed retrospective and prospective studies in the adult setting were evaluated. When limited data existed, expert opinions from pediatric oncologists and radiation oncologists were provided.
Table 1
Items | Specification |
---|---|
Date of search | March 15, 2022 to July 15, 2023 |
Databases and other sources searched | PubMed, MEDLINE, ClinicalTrials.gov |
Search terms used | Radiotherapy; palliative medicine; palliative care; pediatrics; radiosurgery; pediatric oncology; cancer; cancer pain |
Timeframe | 1970–2023 |
Inclusion criteria | English language; prospective and retrospective study type; survey studies; review articles |
Selection process | Selection process was conducted independently with consensus upon peer review of manuscript text by all authors |
Any additional considerations | Augmentation with current and recent clinical trials for pediatric caners |
Principles of palliative RT
External beam RT delivers treatment non-invasively over the course of minutes while the patient lies on a treatment table in a reproducible position (2). Treatments are typically delivered in daily treatments called fractions (3).
RT in the curative setting is typically delivered in conventional fractionation (1.8–2 Gy per treatment delivered over 4–6 weeks on average) to allow for late-responding normal tissues to repair, while higher dose per fraction (hypofractionated) regimens with >2 Gy per day are often used in the palliative setting (3-5). Hypofractionated regimens deliver dose over a shorter duration of time, resulting in a higher biological effectiveness while reducing the number of patient visits (6).
Advancements in RT have led to more conformal radiation with techniques such as intensity-modulated RT (IMRT) (highly modulated form of X-ray treatment) or proton therapy (7,8). However, these treatment techniques can take days to a week or two to plan) and, in the case of protons, are only available in relatively few centers.
Palliative RT must be timely. For patients with rapidly progressing symptoms, that may mean starting radiation within a day. Therefore, 3-dimensional conformation (X-ray) RT (3D-CRT) or electron RT is often the best choice for patients with urgent needs. For patients with slowly progressing disease without escalating symptoms, or in patients with a lesion being treated that appears threatening to but not yet reducing quality of life, radiation can be started in several days to weeks. For patients with less acute and have more refractory histologies, stereotactic radiosurgery or stereotactic body RT (SBRT) may be an option (9,10).
Pediatric specific treatment considerations
The logistics and burden of receiving RT are often greater in children than in adults. Most children’s hospitals are not attached to RT centers, necessitating transportation for urgent or emergent radiation needs. Considerations include medical stability for transport and appropriate staffing should the patient have a medical emergency while undergoing treatment. In cases of high acuity patients, the patient may be accompanied by nurse or physician staff to ensure appropriate pediatric specialty care. Pediatric patients are often treated with general anesthesia for each daily fraction to optimize a reproducible daily set up, and the medical oncologists or medical intensivists are key members of the team in assessing the safety of transporting a patient for treatment.
While daily anesthesia use is necessary in many cases to minimize intra-fraction motion, this increases the potential short- and long-term toxicities associated with RT (11-13). It is emotionally and logistically difficult for patients and their parents, requiring patients to be fasting, or nil per os (NPO) prior to treatment and necessitating longer time in the center. The NPO times can also make it more difficult for patients to maintain nutrition.
Princess Margaret Cancer Centre (Toronto, Canada) has published on the frequency of anesthesia use in pediatric patient for RT. General anesthesia was used in 90% of patients under 3 yo, 28% in patients age 3–6 yo, 1% in patients age 7–11 yo, and <1% in patients ≥12 yo. The threshold at which they transition from the majority of patients needing anesthesia to proceeding without is 4 yo (56.6% for 3 yo, 29.8% for 4 yo) (14). Anesthesia use is higher for each age group at institutions treating a lower volume of pediatric patients, likely due to a lack of awareness of strategies that may be implemented to avoid anesthesia use (15). Strategies may include working with a child-life specialist (16,17), distraction using video technology (18), use of additional educational materials to acclimate the patient to the treatment experience, giving the child a mask to practice with at home prior to simulation and treatment, or allowing comfort objects like a favorite stuffed animal on the treatment table with the patient.
Sometime palliative radiation is delivered in urgent situations where curative therapy is possible. For example, a patient’s disease presentation may include spinal cord compression (SCC) or acute vision loss. In cases such as these, care must be taken with target delineation and treatment planning to minimize long term toxicities of RT. Radiation to growth plates prior to maturation can lead to hypoplasia and asymmetries with significant functional implications as the child develops. Rao et al. present a concise summary with an anatomic road map of growth plates and clinical correlates of radiation-induced growth toxicity (19). Other outcomes of RT in infancy or childhood may include but are not limited to fertility loss, breast hypoplasia, congestive heart failure, pulmonary disease, diabetes mellitus, hypothyroidism, neurocognitive deficits, or secondary malignancies (20-27).
Palliative RT for pediatric leukemia and lymphoma
Leukemia and lymphomas are the most common malignancies in children, accounting for approximately 40% of all pediatric cancers. The primary treatment is chemotherapy, which addresses systemic disease while sparing the potential late effects associated with RT. However, RT continues to play an important role in conditioning for hematopoietic stem cell transplantation, and certain consolidative and palliative scenarios for leukemias and lymphomas, particularly as high-risk, relapsed, and refractory diseases are still very radiosensitive entities (28). In the palliative setting, a multidisciplinary approach to care is crucial in safe and effective management as RT can play an important role in preserving function and relieving symptoms (Table 2).
Table 2
Indication | Histology | Dose and fraction | Expected response |
---|---|---|---|
Superior vena cava syndrome | Any | 20 Gy in 5 | >80% symptomatic relief |
30 Gy in 10 | |||
Ophthalmic manifestations | Any | 18–24 Gy in 10–12 | >85% stabilization/improvement |
Cranial nerve palsy | Any | 10–12 Gy in 5–6 if treating base of skull alone | 80–85% stabilization/improvement |
24 Gy in 12 for whole brain | 80–95% stabilization/improvement | ||
Spinal cord compression | Indolent lymphoma | 4 Gy in 2 | >80% at least partial response |
Leukemia | 24 Gy in 12 | ~95% symptomatic relief and local control | |
High grade non-Hodgkin lymphoma | 30 Gy in 10 | >90% stabilization/improvement | |
Leukemia cutis | Any | 18–26 Gy in 10–13 | 50–90% initial complete response rate |
Symptomatic splenomegaly | Leukemia | 5–10 Gy in 5–10 | 80–90% pain response/reduction in spleen size/cell count improvement |
Non-Hodgkin lymphoma | 20 Gy in 10 or higher | 80–90% reduction in spleen size, local control |
Provider discretion is permitted for dose-fractionation variations based on comprehensive patient evaluation and goals of care.
Indications for palliative RT for leukemias and lymphomas
Superior vena cava syndrome (SVCS)
SVCS refers to a structural compression of the superior vena cava causing outflow obstruction and constellation of symptoms including headache, facial swelling, and dyspnea. The majority of malignancy related SVCS is caused by lung cancer in adults, and is rare among children with leukemias and lymphomas (29). The primary therapy for SVCS secondary to hematologic malignancy is often systemic therapy (30). However, in urgent cases such as airway compromise, combination of corticosteroid with stenting or RT could be considered. While direct robust comparison is lacking, stenting may provide faster venous patency resulting in more immediate and prolonged symptom relief compared to RT (31-33). When RT is indicated with palliative intent, 20 Gy in 5 fractions or 30 Gy in 10 fractions may bring effective symptomatic relief more than 80% of the cases (30,34). For children with lymphomas requiring RT for SVCS and having curative potential, treatment with 1.5–2 Gy per day may be prudent to avoid compromising future RT planning.
Ophthalmic manifestations and cranial palsies
Leukemias and lymphomas may present with ophthalmic manifestations, including eye pain, red eye, and vision loss, particularly since the orbit and the central nervous system (CNS) are potential sanctuary sites from chemotherapy (35). Although retina and posterior globe are the most common sites of ocular abnormality, any ocular structure including the anterior segment or optic nerve pathway can be involved (35). Discussion with the medical oncology team is paramount to evaluate if intrathecal systemic therapy such as high dose methotrexate is warranted as first line versus RT. If there is progression of ocular disease despite adequate CNS-directed systemic therapy, urgent radiation is indicated. Doses of 18–24 Gy in 1.6–2 Gy per fraction have resulted in greater than 85% disease control, although successful disease control with doses low as 8–12 Gy have been reported (36,37). Consultation with ophthalmology and pediatric oncology are required to determine the volume of radiation. At minimum, posterior globe and optic nerve of the affected eye and chiasm are required. However, contralateral posterior globe and optic nerve are often covered due to potential migration of disease or presence of subclinical disease (36). Depending on clinical scenario, the brain and the anterior structures of the eye may be covered as well.
In addition to the optic nerves, any other cranial nerve can be involved by leukemias or lymphomas (38). If cranial palsy is refractory to the appropriate systemic therapy, urgent RT is indicated. Historically, 24 Gy whole brain RT (WBRT) has been commonly used, with response rate of 80–95% (39,40). RT to the base of skull (BOS) alone to doses as low as 10–20 Gy have been used with response rate of 80–85% (38). However, WBRT may be associated with more durable control of CNS disease and prevention of subsequent cranial nerve dysfunction compared to BOS RT alone (41). A lateral opposed parallel pair has the advantage of covering the structures of interest with short planning time, ease of setup, and minimal risk of geographic miss, but more conformal techniques such as IMRT for the whole brain or BOS may be considered to spare as much normal tissue as possible.
SCC
Non-Hodgkin lymphoma (NHL) is the most common histology associated with SCC in pediatric hematology (42,43). Management should involve establishment of diagnosis and initiation of appropriate systemic therapy, including steroids. In cases of refractory disease, RT is indicated to prevent neurological sequelae. Extrapolating from the adult literature, 4 Gy in 2 fractions for indolent lymphoma, 30 Gy in 10 for high-grade NHL, and 24 Gy in 12 for leukemia have been suggested (4,44,45). In contrast to non-hematological malignancy, surgery is not used often due to the chemo and radiosensitive nature of leukemias and lymphomas (46,47). However, surgical decompression may be an option after careful consideration of potential late effects of RT, prognosis, involved disease sites, and the ease of surgical access.
Leukemic infiltration of other organs
Skin is a relatively common site of leukemic infiltration outside of marrow, CNS, and testes. Leukemia cutis (LC) refers to infiltration of leukemic cells into the skin layers, which can cause pruritus, pain, and bleeding (48). In children, they commonly manifest with erythematous or violaceous nodules or papules in the head and lower extremities (48). As LC is often associated with marrow disease, the standard therapy is systemic therapy followed by bone marrow transplant (49). For symptomatic or bleeding lesions, palliative RT can be utilized at any stages of treatment to provide rapid relief of symptoms, although durable local control rate is less than 50% (49,50). Doses between 18–26 Gy in 1.8–2 Gy per fraction have been used, and there is no clear relationship between higher dose and disease response (49-51). Low energy electrons and bolus are typically used to cover the skin lesion adequately. Palliative total skin electron beam therapy (TSEBT) has been used for extensive symptomatic disease.
Splenic irradiation can be performed for symptomatic splenomegaly (i.e., pain, dyspnea, pancytopenia from sequestration, early satiety) in the setting of hematologic malignancy. Daily irradiation of 1 Gy to the whole spleen up to 5–10 Gy can provide significant pain relief or reduction in spleen size with a partial or complete response in 80–90% of patients (52). For NHL treated in the definitive or palliative setting, doses ≥20 Gy resulted in increased durability (median response 35 months vs. 4 months), suggesting consideration of a higher dose for splenic only disease or potentially patients with a limited metastatic burden (53).
Infiltration of other organs is less common. Cases ranging from bowel obstruction and perforation, acute kidney injury, and cardiac tamponade have been described in the literature (54-56). However, extra-CNS organ infiltration of leukemic cells is often asymptomatic and etiologies tend to be multifactorial (56). Supportive care, systemic therapy, and surgical corrections are the mainstays of treatment. Older case reports of heart failure due to cardiac infiltration of acute lymphoblastic leukemia treated with 20 Gy and gastrointestinal intussusception due to CLL infiltration treated with 22 Gy have been published (57,58).
For patients with low-grade disease, “Boom Boom” (2 Gy in 2 fractions) (59,60) and “Big Boom” (4 Gy in 1 fraction) (61) may result in significant relief for most patients with less durability compared to more protracted courses of treatment (60). These very low doses may not preclude the ability to deliver additional RT in the future.
Palliative RT for the CNS
RT is a key treatment for children with cancer who develop CNS pathology. Prior to the initiation of RT, patients should be evaluated for acute findings that need be treated primarily with non-RT approaches. Seizures, hydrocephalus, or focal neurologic deficits are common presenting symptoms. Patients with seizures should be evaluated for increased intracranial pressure; anti-epileptics should also be commenced (62). Patients with hydrocephalus should be evaluated by a neurosurgeon for potential cerebrospinal fluid (CSF) diversion with an extraventricular drain (EVD), endoscopic third ventriculostomy (ETV) or ventriculoperitoneal (VP) shunt. Patients with mass effect or symptomatic brain edema should be treated with dexamethasone prior to RT.
Brain metastases
Brain metastases in children with cancer is rare, but can be a serious presentation of advanced or recurrent disease (63-65). Management depends on whether patients have parenchymal brain metastases or leptomeningeal disease.
Parenchymal brain metastases
Children with a solitary brain metastasis typically follow an approach modelled on adult oncologic care, with initial neurosurgical resection (66), if safe, to achieve diagnostic confirmation and therapeutic relief of mass effect, followed by cavity radiosurgery (67). Children with multiple brain metastases should be evaluated for surgical removal of any symptomatic lesions (if needed), followed by radiosurgery (if small number of lesions) or WBRT (if numerous lesions) (Figure 1). The threshold number of metastases to define treatment with radiosurgery vs. WBRT is unclear in children; however, we have data to suggest treatment up to 15 brain metastases can be safe in adults, but the safety overall depends on the volume of lesions treated (68,69). Consideration of extracranial disease control should be taken into account when determining the role for radiosurgery vs. WBRT.
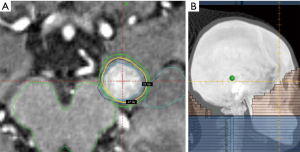
Leptomeningeal disease
Patients with isolated presentation of suspected leptomeningeal disease on magnetic resonance imaging (MRI) brain should have staging investigations completed, including MRI spine and CSF cytology for histologic confirmation, if possible and safe (i.e., no increased intracranial pressure). In children with known extracranial metastases, CSF cytology is not required. Children with intracranial leptomeningeal disease require WBRT with particular attention to include cranial nerve foramina in the skull base and coverage of the optic nerves. Dose prescriptions of 20 Gy in 5 fractions or 30 Gy in 10–12 fractions have been previously used (4).
Children with CNS metastases from rhabdomyosarcoma (70) or neuroblastoma (71,72) may benefit from craniospinal irradiation with conventional daily fractionation (1.8 Gy per day).
SCC
Patients with symptoms of SCC should be evaluated with contrast-enhanced MRI of the entire spine. Children with limited burden of disease outside the spinal column should be emergently referred for neurosurgical evaluation for decompressive surgery, followed by post-operative RT (73,74). Standard dose prescription in this setting is 20 Gy in 5 fractions or 30 Gy in 10 fractions (4).
In children who are not candidates for surgery, definitive RT with 20 Gy in 5 fractions or 30 Gy in 10 fractions should be emergently commenced (4). The role of single-fraction RT for SCC has not been studied in children. Spine SBRT may be suitable for lesions that are not touching the spinal cord, but is contraindicated in the setting of a metastatic lesion causing SCC.
Diffuse intrinsic pontine glioma (DIPG)
Children with DIPG present with acute or subacute onset of cranial neuropathy, ataxia and long-tract signs (75). Lesions typically have a classic radiologic appearance, with T2 hyperintense involvement of the brainstem (76). Neurosurgical consultation for consideration of biopsy may be considered, but is optional if the presentation has no atypical characteristics. If biopsy is undertaken, a histone 3 mutation is commonly found, leading to a pathologic diagnosis of diffuse midline glioma, H3 K27-altered (77).
RT should be commenced urgently, which results in symptomatic improvement in most patients (78). Standard RT prescription is 54 Gy in 30 fractions. An alternate prescription is 39 Gy in 13 fractions if a shorter course of therapy is desired (79-81). If an urgent conformal [IMRT/volumetric-modulated arc therapy (VMAT)] plan cannot be initiated rapidly, patients with progressing symptoms should receive the first few fractions using a 3DCRT plan until a conformal plan can be created (Figure 2).
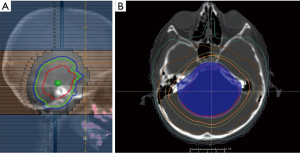
Re-irradiation in the CNS
Clinical situations which may require emergent evaluation for re-irradiation to the CNS include patients with recurrent high-grade glioma (HGG) or DIPG (82,83). Patients should undergo neuroaxis staging (MR spine) to ensure no distant sites of recurrence (84). Children with recurrent HGG can safely receive a course of re-irradiation, which may be associated with improved progression-free survival and overall survival (85,86). Similarly, children with recurrent DIPG can undergo re-irradiation with improvement in symptoms (87) and may be associated with a survival benefit (88). While the optimal dose for re-irradiation is not well defined, with practice variation between institutions, 20 Gy in 10 fractions is a commonly used regimen (79,89).
Palliative RT for pediatric solid tumors
Many pediatric solid tumors are more sensitive to RT than many adult tumors. Yet, practice patterns for treating symptomatic disease in pediatric solid tumor patients vary widely between institutions and treatment centers and across diverse histologies with variable sensitivity to RT (1). Hence, we performed a histology-specific focused review of the literature about the utility of palliative and emergent RT for solid tumors with recommendations summarized in Table 3.
Table 3
Histology | Indication | Dose and fraction | Expected response |
---|---|---|---|
Neuroblastoma | All sites | 20 Gy in 5 | >80% symptomatic relief |
Vision loss | 4.5 Gy in 3 | N/A† | |
Hepatomegaly | 4.5 Gy in 3 | N/A† | |
Osteosarcoma | All sites | 36–45 Gy in 12–15 | >75% response |
SBRT‡ | 40 Gy in 5 | 60% response rate | |
Ewing sarcoma | All sites | 30 Gy in 10 | 84% symptomatic relief |
SBRT‡ | 30–40 Gy in 5 | 100% symptomatic relief | |
Rhabdomyosarcoma | All sites | 30 Gy in 10 | 64% pain relief |
SBRT‡ | 30–35 Gy in 5 | 83% 1-year local control |
Provider discretion is permitted for dose-fractionation variations based on comprehensive patient evaluation and goals of care. †, N/A—often used as a bridge to systemic therapy in the newly diagnosed setting. Limited case numbers to provide expected response rate; ‡, for the properly selected patient (KPS >50–60%, size ≤5 cm, non-emergent). SBRT, stereotactic body radiotherapy; KPS, Karnofsky Performance Status; N/A, not available.
Neuroblastoma
Neuroblastoma is the most common extracranial tumor in children. Treatment routinely involves a multimodality approach given the high disease burden and metastatic potential associated with this pathology. Although RT is commonly used for consolidation in the treatment of high-risk neuroblastoma to the post-resection tumor bed and up to five chemoimmunotherapy refractory metastatic sites, no clear clinical guidelines have established a radiation regimen for palliative treatment in metastatic disease.
Extrapolating from treatment in the upfront setting, excellent local control was demonstrated on ANBL0532 with 21.6 Gy with no additional improvement in local control seen with dose-escalation to 36 Gy in the setting of gross residual disease >1 cm3, highlighting the radiosensitive nature of this disease (90-93). Moreover, dose reduction to 18 Gy for local control to the primary site may be sufficient and warrants validation on a multi-institutional trial (94). For metastatic sites, the strongest prognostic factor for local recurrence is persistence after induction chemotherapy, supporting the consolidative role of RT which results in LC >90% with doses of 21–24 Gy (90,91,93).
Retrospective studies in the palliative setting demonstrate similar efficacy with modest RT doses for local control and palliation. A French series by Caussa et al. evaluated the impact of dose-response for palliative RT among 34 patients (69 metastatic sites) with metastatic high-risk neuroblastoma with overall response rate measured by a composite endpoint of pain relief or >25% reduction in tumor volume (95). Soft tissue metastases treated to a median dose of 20 Gy (range, 8–36 Gy) appeared to be the most responsive with 84.2% response rate, which increased to 100% when doses ≥15 Gy were used. Bone (median dose 16.5 Gy) and CNS metastases (median dose 15 Gy) appeared to respond less (63.2% and 44%, respectively), with a trend toward improved response with higher doses ≥20 Gy (81.2% vs. 50%) for bone lesions. Similarly, Lazarev et al. reported 1-year local control of 82% in 50 patients with neuroblastoma treated with a short hypofractionated schedule of schedule of >1 but ≤5 fractions at ≥3 Gy; albeit median total dose of 24 Gy in 5 fractions was histology agnostic in this solid tumor cohort study raising the question of the dose received by the neuroblastoma patients specifically (96).
Recent protocols support the use of emergent RT at diagnosis to sites of life-threatening or functional-impairing disease, such as vision loss and hepatomegaly resulting in severe respiratory distress using low doses of 3 fractions at 1.5 Gy each (4.5 Gy total). For vision symptoms, evaluation by pediatric ophthalmology to correlate objective vision symptoms with imaging related findings can be critical as the physical exam in this often very young age group can be challenging. As per ANBL 1531, these sites do not require consolidation at time of RT for high-risk patients if these metastatic sites do not otherwise meet the criteria for requiring treatment.
While the prognosis remains poor in this setting, the meaningful benefit with modest doses required for palliation may outweigh the risk of long-term treatment related morbidity (26,27).
Osteosarcoma
Osteosarcomas are primary malignant tumors of the bone that are managed with systemic therapy and usually surgery for local control. In pediatric patients with metastatic osteosarcoma, RT has been shown as an effective method of symptom control despite being radioresistant. In 1992, Lombardi et al. showed a complete or partial radiologic response in 81% of symptomatic lesions treated with a total dose of 36 Gy (3 weekly fractions of 6 Gy over 2 weeks) (97). Late toxicities observed included fibrosis, pathologic fracture, and perioperative wound infection. More recent data from Chen et al. in 2020 supported the use of higher dose to gain longer symptom relief with minimal toxicity (98). In this study, symptomatic improvement was seen in 75% of cases using the median equivalent dose in 2 Gy fractions (EQD2) of 40 Gy (range, 20–60.4 Gy) with a median number of fractions per course of 15 (98). Median time to symptom improvement was 15.5 days (range, 5–39 days) with median time to progression at 12.9 months. Similar outcomes of complete or partial responses in 61% of lesions treated has been demonstrated by Lazarev et al. (96).
In addition to conventional RT, SBRT has been used successfully for symptomatic control of osteosarcoma. In 2014, Brown et al. documented a 60% response rate for patients treated with symptomatic lesions at a median SBRT dose of 40 Gy in 5 fractions (range, 30–60 Gy in 1–10 fractions) (9). Similar response rates for osteosarcoma of 54% was demonstrated by Rahn and colleagues using a median dose per fraction and fractionation of 3 Gy and 10, respectively (i.e., 30 Gy in 10 fractions) (28). No Radiation Therapy Oncology Group (RTOG) grade 3 acute or late toxicities were observed (28).
Ewing sarcoma
Ewing sarcoma is an aggressive soft tissue tumor of adolescents and young adults, comprising approximately 5% of all soft tissue sarcomas with median age at diagnosis of 15 years (99). Ewing sarcoma and rhabdomyosarcoma are considered more chemotherapy and radiosensitive histologies compared to osteosarcoma and other soft tissue sarcomas.
In the upfront metastatic Ewing sarcoma setting, given long-term cure is possible, a local control strategy applied to primary and metastatic disease sites can prolong event-free survival (100). Effort for local control at week 13 of chemotherapy is recommended as well as metastatic site consolidation at the end of therapy (100,101). If metastatic sites are too numerous to treat, attention is focused on treatment refractory sites and/or sites that if progression were to occur could result in functional impairment.
If a patient presents with functional impairment, such as SCC, emergent initiation of steroids, systemic therapy and/or consideration of urgent RT is warranted since rapid response can be observed (102). If emergent RT is necessary at diagnosis, initiation of RT with conventional fractionation (1.8 Gy per day) with subsequent conversion to a definitive dose RT plan as a continuous course with the inclusion of chemotherapy is recommended for durable local control.
In Ewing sarcoma, the intent of RT for metastatic sites for palliation versus cure depends on multiple factors. In 2006, Koontz et al. described a 55% complete response rate and 29% partial response rate for pediatric patients (median age: 11.6 yo) undergoing palliative radiation for metastatic Ewing sarcoma using an average dose of 30 Gy (range, 4.5–68.5 Gy) (103). In the study previously discussed by Rahn et al. [2015], higher response rates (76%) were seen for pediatric patients treated with a median dose per fraction of 3 Gy (range, 1.5–20 Gy) over a median of 10 fraction (range, 1–28 fractions) (i.e., 30 Gy in 10 fractions) (28). Like osteosarcoma, the use of SBRT has shown considerable effectiveness in symptomatic relief with an acceptable side effect profile. Brown et al. showed a 100% response rate for symptomatic Ewing sarcoma metastases treated with a median SBRT dose of 40 Gy given in 5 fractions (range, 30–60 Gy in 1–10 fractions) (9).
Rhabdomyosarcoma
Rhabdomyosarcoma is the most common soft-tissue sarcoma in the pediatric population with survival rates of those presenting with metastatic disease still poor at around 30% (104). A similar approach to Ewing sarcoma described in detail above is taken for metastatic rhabdomyosarcoma.
Parameningeal rhabdomyosarcomas are at risk for leptomeningeal disease, and careful attention at initial diagnosis with adequate staging (e.g., MRI craniospinal axis and lumbar puncture) and consideration of restaging if a patient is presenting with failure-to-thrive and symptoms (e.g., nausea, neurologic symptoms) beyond what would be anticipated for the current known extent of disease (70). Treatment for leptomeningeal disease may benefit from consideration of craniospinal irradiation to a median dose of 36 Gy (range, 18–36 Gy) and/or WBRT to a median dose of 30 Gy (range, 6–41.4 Gy) with careful attention to prior areas of irradiation in regard to cumulative dose (70).
Compared to Ewing sarcoma, even more limited data exists regarding the optimal dose fractionation for the palliative treatment of rhabdomyosarcoma in the pediatric population. As discussed previously, Rahn et al. for pediatric rhabdomyosarcoma patients a 64% response rate with a median dose-fraction regimen of 30 Gy in 10 fractions (28). Skamene and colleagues reported excellent (100%) local control for all 12 metastatic sites treated with definitive dose RT (41.4–50.4 Gy in 1.8 Gy fractions) with minimal treatment related toxicity (105).
Parsai et al. reported promising data in regard to the use of SBRT for pediatric, adolescent and young adult patients with sarcoma, including rhabdomyosarcoma, Ewing sarcoma, osteosarcoma, and other soft tissue sarcomas with excellent local control of 88.3% at 6 months, which was modestly reduced to 83% at 12 months for those surviving longer than 1 year (106). Importantly, the majority of local failures were in-field (60%), while the minority were marginal failures (40%), emphasizing the importance to study the most effective dose (for in-field failures) and ensuring adequate treatment volumes (for marginal failures).
Symptom management
A principle of palliative RT is to design a treatment plan to balance efficacy and durability of response with the intent of keeping risk of acute and late toxicities to a minimum. In many cases while the effects may be modest, these events may not be avoided entirely, and proactive symptom management may help reduce the impact (Table 4). The timeline of acute symptom development is often a gradual onset with the course of RT, peaking at the culmination or up to 1 week after treatment with gradual improvement with resolution of most effects seen by 4–6 weeks.
Table 4
Treatment site | Acute side effects | Recommendations |
---|---|---|
Brain | Nausea | 5-HT3 antagonist, scopolamine patch, lorazepam, olanzapine, or dexamethasone for refractory nausea |
Headache | Tylenol/NSAIDs, opioids, dexamethasone | |
Seizures | Prophylactic antiepileptics, dexamethasone | |
Swelling/somnolence | Dexamethasone | |
Dermatitis | Emollients, topical steroids | |
Head & neck | Mucositis, esophagitis | Viscous lidocaine (as a compound: equal parts mixed 1:1:1-lidocaine, diphenhydramine/benadryl, aluminum hydroxide and magnesium hydroxide suspension/Maalox) |
Xerostomia | frequent sips of water, sodium bicarbonate rinses | |
Dermatitis | Emollients, topical steroids | |
Thrush | Nystatin, fluconazole | |
Thickened secretions | Guaifenesin, papaya juice, meat tenderizer | |
Otitis-media or externa | Antibiotic/steroid drops | |
Dry eye | Artificial tears (daytime), eye ointment (nighttime) | |
Chest/thorax | Esophagitis | PPI or antacid, dietary adjustments |
Pericarditis | NSAIDs, or dexamethasone with taper | |
Pneumonitis | Inhaled steroids for minimal symptoms, dexamethasone with taper for more severe | |
Dermatitis | Emollients and topical steroids | |
Upper abdomen | Nausea | 5-HT3 antagonists |
Anorexia | Cyproheptadine, dronabinol (Marinol) | |
Gastritis | Carafate, antacids, PPIs | |
Dermatitis | Emollients, topical steroids | |
Lower abdomen/pelvis | Cystitis | Phenazopyridine (Pyridium), dietary adjustments (low acidity, caffeine, spicy food, carbonated beverages) |
Enteritis | Loperamide | |
Dermatitis | Emollients, topical steroids | |
Extremity/bone | Pain flare | Tylenol, NSAIDs, opioids, dexamethasone |
Dermatitis | Emollients, topical steroids | |
Fracture | Pain medications or surgical stabilization |
NSAIDs, nonsteroidal anti-inflammatory drugs; PPI, proton pump inhibitor.
Radiation fatigue, akin to “participating in extracurricular activities” is best managed with gentle exercise and added rest.
Radiation dermatitis can result in folliculitis, dry skin, and/or red/peeling skin. This is typically managed with topical emollients twice a day, avoiding application 2 hours prior to treatment to minimize risk of intensification of radiation, and topical diphenhydramine (Benadryl) and/or hydrocortisone cream for pruritus.
Nausea is common, particularly with treatment fields near the brain, thorax, and gastrointestinal tract. As opposed to other acute effects of radiation, nausea can occur following the first treatment. Prophylactic use of 5-HT3 antagonists, such as ondansetron, dosed 1 hour prior to treatment is typically recommended. Lorazepam can mitigate nausea with an anxiety component, and for younger kids to minimize motion. A scopolamine patch can offer a non-sedating alternative. Olanzapine can help if there is an anorexia component. A short course of dexamethasone can be used in cases of refractory nausea.
Palliative radiation to the brain can cause additional acute effects like alopecia, somnolence, as well as headaches and swelling, which could lead to worsening or new neurological symptoms or seizures. Dexamethasone is useful to control these symptoms.
Esophagitis can be managed with dietary adjustments, such as avoiding dry, sharp, or acidic foods. For lower esophagitis or gastritis, a proton pump inhibitor (PPI) or antacids can offer significant relief. For proximal esophagitis or mucositis, a compounded lidocaine solution using a combination of 1:1:1 diphenhydramine, aluminum hydroxide and magnesium hydroxide suspension (Maalox), and viscous lidocaine offers symptomatic relief. If symptoms persist, care should be taken to rule out esophageal candidiasis.
Treatment fields involving the lower abdomen or pelvis can cause enteritis or cystitis. Acute diarrhea can be treated with dietary changes (constipation-promoting diet), along with loperamide as needed (assuming an infectious etiology, such as Clostridium difficle infection, is ruled out) (107). Urinary discomfort can be managed by dietary changes (reducing caffeine, acidity, carbonated beverages), and phenazopyridine (Pyridium).
Palliative radiation is often used to treat bony pain caused by disease, but treating these bony sites in the minority of cases can result in an inflammatory pain flare that can last hours to up to 7 days. This can be treated with analgesics such as opioids, nonsteroidal anti-inflammatory drugs (NSAIDs) (if clinically safe to utilize) or a short pulse of steroids (96,108,109).
Communication with patients and families
High-quality communication is key to providing the best possible care for patients and their families. Patients and caregivers report greater prognostic understanding, increased confidence in their decisions, and improved quality of life when clinicians practice direct and compassionate communication about prognosis and anticipated symptoms (110-112). Children with cancer and their caregivers also want direct, honest, and empathic discussions with their clinicians, even when the information is difficult or distressing (113). Finally, patients and families who are part of such discussions report increased peace of mind and hopefulness, greater trust in their clinicians, and ultimately, less decisional regret (111,114). These outcomes are especially important when patient symptom burden may be high and/or likelihood of cure may be low.
And yet, communication in the setting of palliative and emergent RT for children with cancer can be challenging. Formal communication training for oncology clinicians is limited, leaving many clinicians underprepared to facilitate these complicated and emotionally challenging conversations. Indeed, the bulk of clinician communication training is focused on delivery of treatment options (i.e., “let’s talk about the treatment option of RT”) without the concurrent focus on that treatment’s outcomes (i.e., “this treatment may alleviate some of your symptoms, and is not expected to cure your cancer”). Additionally, they may feel that by discussing “bad news” with patients and families, they are depriving them of a critical resource when they need it most: hope (115,116); nearly half of pediatric oncology clinicians equate these discussions with a “personal failure” (117). Perhaps for these reasons, a quarter of parents report unmet prognostic information needs regarding their child’s cancer (118), most feel additional distress with their decision-making, and many regret missed opportunities to talk to their child and/or optimize their child’s overall quality of life (112,116,119-121).
To bridge this gap, clinicians must model consistent, evidence-based communication strategies. Many of the core principles in adult oncology and palliative care communication science also apply to the care of children with cancer and their families. These include the primary roles for communication in this setting: to exchange information, to foster relationships, and to provide a forum for decision making (122). It is important to begin conversations related to goals of care early and revisit them often, to expect and validate emotion, and to elicit values and preferences to inform treatment decisions. There are also aspects of communication that are unique to pediatric oncology. For example, clinicians must navigate how to include children in these discussions in developmentally appropriate ways, accommodate individualized family dynamics, and balance caregiver and child autonomy in terms of decision making (123).
Pragmatic communication support guides for oncology providers exist (122), and ongoing research in the pediatric palliative care and oncology fields is helping to define key components of high-quality communication (124). Establishing a human connection through affection or remembering things important to patients and families can help build a framework of trust. Using NURSE statements to respond to patient and family emotion (Naming the apparent emotion, statements of Understanding or normalization of that emotion, paying Respect to parent roles and values, pledging Support of their child, family, and the shared decision-making process, and Exploring their thoughts, values, hopes and worries) can support the family and clinician through a difficult conversation (125). Families of children with advanced cancer have also identified inclusivity, humor, alliance, and feeling as if their clinician is partnering with them as facilitators of a therapeutic communication relationship (124).
Clinicians caring for children receiving palliative or emergent RT play a critical role in supporting decisions and clinical care at a time when such things have profound impacts on both immediate and long-term well-being of the entire family unit. Clinicians rely on patients and caregivers to disclose their most personal needs, hopes, and values; and they depend on the clinician to provide honest, empathetic, and complete information. This two-way partnership only works through thoughtful and skillful communication. When therapeutic relationships are built upon a foundation of strong communication, clinicians are more likely to be able to provide the kind of high-quality, compassionate, goal-concordant care that all patients deserve.
Conclusions
We have demonstrated the valuable benefit that palliative RT may have across all major pediatric tumor types along with the tools for how to empower the decision making of our patients and their families during this precious time. Advancements in the technology of RT continually allow for more rapid planning and increased precision and conformality in hope to widen the therapeutic threshold of maximizing efficacy and durability of response with reduced side effects. Yet, technological advancements alone are insufficient to best serve pediatric patients. Rather, there is a need to identify the lowest effective dose over the shortest length of time to reduce the logistical impact on quality of life and minimize risk of long-term effects. Coordination with the multi-disciplinary treatment team is critical to minimize delays in systemic therapy when employing palliative RT, and consideration of minimizing overlapping toxicities with systemic therapy and RT.
For pediatric patients, our literature review highlights the absence of prospective data for the use of palliative RT along with histology-specific data. There is an urgent need for prospective investigation of the value of palliative RT with involvement and careful attention to the patient and caregiver reported outcomes.
Acknowledgments
Funding: None.
Footnote
Provenance and Peer Review: This article was commissioned by the editorial office, Annals of Palliative Medicine, for the series “Radiotherapy for Oncologic Emergencies”. The article has undergone external peer review.
Reporting Checklist: The authors have completed the Narrative Review reporting checklist. Available at https://apm.amegroups.com/article/view/10.21037/apm-23-505/rc
Peer Review File: Available at https://apm.amegroups.com/article/view/10.21037/apm-23-505/prf
Conflicts of Interest: All authors have completed the ICMJE uniform disclosure form (available at https://apm.amegroups.com/article/view/10.21037/apm-23-505/coif). The series “Radiotherapy for Oncologic Emergencies” was commissioned by the editorial office without any funding or sponsorship. D.S.T. has received grant funding from the National Institutes of Health, Princess Margaret Cancer Foundation, and Brain Tumor Foundation. In addition, D.S.T. receives consulting fees CADH and NEED including stock options from NEED. D.S.T. has received support from Elekta AB and Mevion Medical systems in 2022. D.S.T. serves on the ASTRO Education Committee. All of D.S.T.’s disclosures are unrelated to this work. S.K.S. served as an unpaid Guest Editor of the series. The authors have no other conflicts of interest to declare.
Ethical Statement: The authors are accountable for all aspects of the work in ensuring that questions related to the accuracy or integrity of any part of the work are appropriately investigated and resolved.
Open Access Statement: This is an Open Access article distributed in accordance with the Creative Commons Attribution-NonCommercial-NoDerivs 4.0 International License (CC BY-NC-ND 4.0), which permits the non-commercial replication and distribution of the article with the strict proviso that no changes or edits are made and the original work is properly cited (including links to both the formal publication through the relevant DOI and the license). See: https://creativecommons.org/licenses/by-nc-nd/4.0/.
References
- Rao AD, Chen Q, Ermoian RP, et al. Practice patterns of palliative radiation therapy in pediatric oncology patients in an international pediatric research consortium. Pediatr Blood Cancer 2017; [Crossref] [PubMed]
- Berman AT, Plastaras JP, Vapiwala N. Radiation oncology: a primer for medical students. J Cancer Educ 2013;28:547-53. [Crossref] [PubMed]
- Bernier J, Hall EJ, Giaccia A. Radiation oncology: a century of achievements. Nat Rev Cancer 2004;4:737-47. [Crossref] [PubMed]
- Tsang DS, Vargo JA, Goddard K, et al. Palliative radiation therapy for children with cancer. Pediatr Blood Cancer 2021;68:e28292. [Crossref] [PubMed]
- Chandra RA, Keane FK, Voncken FEM, et al. Contemporary radiotherapy: present and future. Lancet 2021;398:171-84. [Crossref] [PubMed]
- Grimm J, Marks LB, Jackson A, et al. High Dose per Fraction, Hypofractionated Treatment Effects in the Clinic (HyTEC): An Overview. Int J Radiat Oncol Biol Phys 2021;110:1-10. [Crossref] [PubMed]
- Mohan R, Grosshans D. Proton therapy - Present and future. Adv Drug Deliv Rev 2017;109:26-44. [Crossref] [PubMed]
- Taylor A, Powell ME. Intensity-modulated radiotherapy--what is it? Cancer Imaging 2004;4:68-73. [Crossref] [PubMed]
- Brown LC, Lester RA, Grams MP, et al. Stereotactic body radiotherapy for metastatic and recurrent ewing sarcoma and osteosarcoma. Sarcoma 2014;2014:418270. [Crossref] [PubMed]
- Abel S, Lee S, Ludmir EB, et al. Principles and Applications of Stereotactic Radiosurgery and Stereotactic Body Radiation Therapy. Hematol Oncol Clin North Am 2019;33:977-87. [Crossref] [PubMed]
- Banerjee P, Rossi MG, Anghelescu DL, et al. Association Between Anesthesia Exposure and Neurocognitive and Neuroimaging Outcomes in Long-term Survivors of Childhood Acute Lymphoblastic Leukemia. JAMA Oncol 2019;5:1456-63. [Crossref] [PubMed]
- Verma V, Beethe AB, LeRiger M, et al. Anesthesia complications of pediatric radiation therapy. Pract Radiat Oncol 2016;6:143-54. [Crossref] [PubMed]
- De Francisci G, Papasidero AE, Spinazzola G, et al. Update on complications in pediatric anesthesia. Pediatr Rep 2013;5:e2. [Crossref] [PubMed]
- Ntoukas SM, Ritchie T, Awrey S, et al. Minimizing General Anesthetic Use in Pediatric Radiation Therapy. Pract Radiat Oncol 2020;10:e159-65. [Crossref] [PubMed]
- Holt DE, Hiniker SM, Kalapurakal JA, et al. Improving the Pediatric Patient Experience During Radiation Therapy-A Children's Oncology Group Study. Int J Radiat Oncol Biol Phys 2021;109:505-14. [Crossref] [PubMed]
- Scott MT, Todd KE, Oakley H, et al. Reducing Anesthesia and Health Care Cost Through Utilization of Child Life Specialists in Pediatric Radiation Oncology. Int J Radiat Oncol Biol Phys 2016;96:401-5. [Crossref] [PubMed]
- Grissom S, Boles J, Bailey K, et al. Play-based procedural preparation and support intervention for cranial radiation. Support Care Cancer 2016;24:2421-7. [Crossref] [PubMed]
- Gutkin PM, Skinner L, Jiang A, et al. Feasibility of the Audio-Visual Assisted Therapeutic Ambience in Radiotherapy (AVATAR) System for Anesthesia Avoidance in Pediatric Patients: A Multicenter Trial. Int J Radiat Oncol Biol Phys 2023;117:96-104. [Crossref] [PubMed]
- Rao AD, Ladra M, Dunn E, et al. A Road Map for Important Centers of Growth in the Pediatric Skeleton to Consider During Radiation Therapy and Associated Clinical Correlates of Radiation-Induced Growth Toxicity. Int J Radiat Oncol Biol Phys 2019;103:669-79. [Crossref] [PubMed]
- Green DM, Lange JM, Peabody EM, et al. Pregnancy outcome after treatment for Wilms tumor: a report from the national Wilms tumor long-term follow-up study. J Clin Oncol 2010;28:2824-30. [Crossref] [PubMed]
- Fürst CJ, Lundell M, Ahlbäck SO, et al. Breast hypoplasia following irradiation of the female breast in infancy and early childhood. Acta Oncol 1989;28:519-23. [Crossref] [PubMed]
- Green DM, Grigoriev YA, Nan B, et al. Congestive heart failure after treatment for Wilms' tumor: a report from the National Wilms' Tumor Study group. J Clin Oncol 2001;19:1926-34. [Crossref] [PubMed]
- Green DM, Lange JM, Qu A, et al. Pulmonary disease after treatment for Wilms tumor: a report from the national wilms tumor long-term follow-up study. Pediatr Blood Cancer 2013;60:1721-6. [Crossref] [PubMed]
- de Vathaire F, El-Fayech C, Ben Ayed FF, et al. Radiation dose to the pancreas and risk of diabetes mellitus in childhood cancer survivors: a retrospective cohort study. Lancet Oncol 2012;13:1002-10. [Crossref] [PubMed]
- Lange JM, Takashima JR, Peterson SM, et al. Breast cancer in female survivors of Wilms tumor: a report from the national Wilms tumor late effects study. Cancer 2014;120:3722-30. [Crossref] [PubMed]
- Yu JI, Lim DH, Jung SH, et al. The effects of radiation therapy on height and spine MRI characteristics in children with neuroblastoma. Radiother Oncol 2015;114:384-8. [Crossref] [PubMed]
- Cohen LE, Gordon JH, Popovsky EY, et al. Late effects in children treated with intensive multimodal therapy for high-risk neuroblastoma: high incidence of endocrine and growth problems. Bone Marrow Transplant 2014;49:502-8. [Crossref] [PubMed]
- Rahn DA 3rd, Mundt AJ, Murphy JD, et al. Clinical outcomes of palliative radiation therapy for children. Pract Radiat Oncol 2015;5:183-7. [Crossref] [PubMed]
- Nossair F, Schoettler P, Starr J, et al. Pediatric superior vena cava syndrome: An evidence-based systematic review of the literature. Pediatr Blood Cancer 2018;65:e27225. [Crossref] [PubMed]
- Perez-Soler R, McLaughlin P, Velasquez WS, et al. Clinical features and results of management of superior vena cava syndrome secondary to lymphoma. J Clin Oncol 1984;2:260-6. [Crossref] [PubMed]
- Fagedet D, Thony F, Timsit JF, et al. Endovascular treatment of malignant superior vena cava syndrome: results and predictive factors of clinical efficacy. Cardiovasc Intervent Radiol 2013;36:140-9. [Crossref] [PubMed]
- Nicholson AA, Ettles DF, Arnold A, et al. Treatment of malignant superior vena cava obstruction: metal stents or radiation therapy. J Vasc Interv Radiol 1997;8:781-8. [Crossref] [PubMed]
- Rowell NP, Gleeson FV. Steroids, radiotherapy, chemotherapy and stents for superior vena caval obstruction in carcinoma of the bronchus: a systematic review. Clin Oncol (R Coll Radiol) 2002;14:338-51. [Crossref] [PubMed]
- Ahmann FR. A reassessment of the clinical implications of the superior vena caval syndrome. J Clin Oncol 1984;2:961-9. [Crossref] [PubMed]
- Talcott KE, Garg RJ, Garg SJ. Ophthalmic manifestations of leukemia. Curr Opin Ophthalmol 2016;27:545-51. [Crossref] [PubMed]
- Somervaille TC, Hann IM, Harrison G, et al. Intraocular relapse of childhood acute lymphoblastic leukaemia. Br J Haematol 2003;121:280-8. [Crossref] [PubMed]
- LaRiviere MJ, Avery RA, Dolan JG, et al. Emergent Radiation for Leukemic Optic Nerve Infiltration in a Child Receiving Intrathecal Methotrexate. Pract Radiat Oncol 2019;9:226-30. [Crossref] [PubMed]
- Ingram LC, Fairclough DL, Furman WL, et al. Cranial nerve palsy in childhood acute lymphoblastic leukemia and non-Hodgkin's lymphoma. Cancer 1991;67:2262-8. [Crossref] [PubMed]
- Gray JR, Wallner KE. Reversal of cranial nerve dysfunction with radiation therapy in adults with lymphoma and leukemia. Int J Radiat Oncol Biol Phys 1990;19:439-44. [Crossref] [PubMed]
- Ha CS, Chung WK, Koller CA, et al. Role of radiation therapy to the brain in leukemic patients with cranial nerve palsies in the absence of radiological findings. Leuk Lymphoma 1999;32:497-503. [Crossref] [PubMed]
- Walker GV, Shihadeh F, Kantarjian H, et al. Comprehensive craniospinal radiation for controlling central nervous system leukemia. Int J Radiat Oncol Biol Phys 2014;90:1119-25. [Crossref] [PubMed]
- Pollono D, Tomarchia S, Drut R, et al. Spinal cord compression: a review of 70 pediatric patients. Pediatr Hematol Oncol 2003;20:457-66. [Crossref] [PubMed]
- Lewis DW, Packer RJ, Raney B, et al. Incidence, presentation, and outcome of spinal cord disease in children with systemic cancer. Pediatrics 1986;78:438-43. [Crossref] [PubMed]
- Yahalom J, Dabaja BS, Ricardi U, et al. ILROG emergency guidelines for radiation therapy of hematological malignancies during the COVID-19 pandemic. Blood 2020;135:1829-32. [Crossref] [PubMed]
- Bakst R, Wolden S, Yahalom J. Radiation therapy for chloroma (granulocytic sarcoma). Int J Radiat Oncol Biol Phys 2012;82:1816-22. [Crossref] [PubMed]
- Rades D, Conde-Moreno AJ, Cacicedo J, et al. Radiation Therapy Alone Provides Excellent Outcomes for Spinal Cord Compression from Vertebral Lymphoma. Anticancer Res 2016;36:3081-3. [PubMed]
- Kaloostian PE, Yurter A, Zadnik PL, et al. Current paradigms for metastatic spinal disease: an evidence-based review. Ann Surg Oncol 2014;21:248-62. [Crossref] [PubMed]
- Andriescu EC, Coughlin CC, Cheng CE, et al. Pediatric leukemia cutis: A case series. Pediatr Dermatol 2019;36:658-63. [Crossref] [PubMed]
- Bakst R, Yahalom J. Radiation therapy for leukemia cutis. Pract Radiat Oncol 2011;1:182-7. [Crossref] [PubMed]
- Elsayad K, Oertel M, Haverkamp U, et al. The effectiveness of radiotherapy for leukemia cutis. J Cancer Res Clin Oncol 2017;143:851-9. [Crossref] [PubMed]
- Bakst RL, Dabaja BS, Specht LK, et al. Use of Radiation in Extramedullary Leukemia/Chloroma: Guidelines From the International Lymphoma Radiation Oncology Group. Int J Radiat Oncol Biol Phys 2018;102:314-9. [Crossref] [PubMed]
- Zaorsky NG, Williams GR, Barta SK, et al. Splenic irradiation for splenomegaly: A systematic review. Cancer Treat Rev 2017;53:47-52. [Crossref] [PubMed]
- Hubbeling H, Imber BS, Wijetunga NA, et al. Safety and Efficacy of Splenic Irradiation for Non-Hodgkin Lymphoma. Blood 2022;140:9538-9. [Crossref]
- Majumder A, Misra S, Kumar V, et al. Intussusception in a child with Acute Lymphoblastic Leukemia: a remarkable presentation with literature review - a case report. J Egypt Natl Canc Inst 2021;33:18. [Crossref] [PubMed]
- Goh TS, LeQuesne GW, Wong KY. Severe infiltration of the kidneys with ultrasonic abnormalities in acute lymphoblastic leukemia. Am J Dis Child 1978;132:1204-5. [PubMed]
- Luo Z, Cheng J, Wang Y. Cardiac Infiltration as the First Manifestation of Acute Lymphoblastic Leukemia: A Systematic Review. Front Oncol 2022;12:805981. [Crossref] [PubMed]
- HADDY TB. CARDIAC IRRADIATION IN CHILDHOOD LEUKEMIA. Am J Dis Child 1964;108:559-65. [PubMed]
- Fromke VL, Weber LW. Extensive leukemic infiltration of the gastrointestinal tract in chronic lymphosarcoma cell leukemia. Am J Med 1974;56:879-82. [Crossref] [PubMed]
- Imber BS, Chau KW, Lee J, et al. Excellent response to very-low-dose radiation (4 Gy) for indolent B-cell lymphomas: is 4 Gy suitable for curable patients? Blood Adv 2021;5:4185-97. [Crossref] [PubMed]
- Hoskin PJ, Kirkwood AA, Popova B, et al. 4 Gy versus 24 Gy radiotherapy for patients with indolent lymphoma (FORT): a randomised phase 3 non-inferiority trial. Lancet Oncol 2014;15:457-63. [Crossref] [PubMed]
- Hajj C, Lee J, Fregonese B, et al. “Big Boom” Radiation Treatment (RT) of 4 Gy Single Fraction: Is It an Acceptable Alternative to “Boom Boom” Radiation Treatment 2 Gyx2 Fractions for Non-Hodgkin Lymphomas (NHL)? Int J Radiat Oncol Biol Phys 2021;111:2620. [Crossref]
- Partap S, Fisher PG. Levetiracetam for seizures in children with brain tumors and other cancers. Pediatr Blood Cancer 2009;52:288-9. [Crossref] [PubMed]
- Suki D, Khoury Abdulla R, Ding M, et al. Brain metastases in patients diagnosed with a solid primary cancer during childhood: experience from a single referral cancer center. J Neurosurg Pediatr 2014;14:372-85. [Crossref] [PubMed]
- Bouffet E, Doumi N, Thiesse P, et al. Brain metastases in children with solid tumors. Cancer 1997;79:403-10. [Crossref] [PubMed]
- Kumar RS, Rotondo RL. Pediatric Brain Metastases. In: Mahajan A, Paulino A. editors. Radiation Oncology for Pediatric CNS Tumors. Cham: Springer International Publishing; 2018:393-410.
- Patchell RA, Tibbs PA, Walsh JW, et al. A randomized trial of surgery in the treatment of single metastases to the brain. N Engl J Med 1990;322:494-500. [Crossref] [PubMed]
- Brown PD, Ballman KV, Cerhan JH, et al. Postoperative stereotactic radiosurgery compared with whole brain radiotherapy for resected metastatic brain disease (NCCTG N107C/CEC·3): a multicentre, randomised, controlled, phase 3 trial. Lancet Oncol 2017;18:1049-60. [Crossref] [PubMed]
- Yamamoto M, Sato Y, Higuchi Y, et al. A Cohort Study of Stereotactic Radiosurgery Results for Patients With 5 to 15 Versus 2 to 4 Brain Metastatic Tumors. Adv Radiat Oncol 2019;5:358-68. [Crossref] [PubMed]
- Milano MT, Grimm J, Niemierko A, et al. Single- and Multifraction Stereotactic Radiosurgery Dose/Volume Tolerances of the Brain. Int J Radiat Oncol Biol Phys 2021;110:68-86. [Crossref] [PubMed]
- De B, Kinnaman MD, Wexler LH, et al. Central nervous system relapse of rhabdomyosarcoma. Pediatr Blood Cancer 2018; [Crossref] [PubMed]
- Croog VJ, Kramer K, Cheung NK, et al. Whole neuraxis irradiation to address central nervous system relapse in high-risk neuroblastoma. Int J Radiat Oncol Biol Phys 2010;78:849-54. [Crossref] [PubMed]
- Luo LY, Kramer K, Cheung NV, et al. Reduced-dose craniospinal irradiation for central nervous system relapsed neuroblastoma. Pediatr Blood Cancer 2020;67:e28364. [Crossref] [PubMed]
- Fehlings MG, Nater A, Tetreault L, et al. Survival and Clinical Outcomes in Surgically Treated Patients With Metastatic Epidural Spinal Cord Compression: Results of the Prospective Multicenter AOSpine Study. J Clin Oncol 2016;34:268-76. [Crossref] [PubMed]
- Patchell RA, Tibbs PA, Regine WF, et al. Direct decompressive surgical resection in the treatment of spinal cord compression caused by metastatic cancer: a randomised trial. Lancet 2005;366:643-8. [Crossref] [PubMed]
- Cohen KJ, Jabado N, Grill J. Diffuse intrinsic pontine gliomas-current management and new biologic insights. Is there a glimmer of hope? Neuro Oncol 2017;19:1025-34. [Crossref] [PubMed]
- Cooney TM, Cohen KJ, Guimaraes CV, et al. Response assessment in diffuse intrinsic pontine glioma: recommendations from the Response Assessment in Pediatric Neuro-Oncology (RAPNO) working group. Lancet Oncol 2020;21:e330-6. [Crossref] [PubMed]
- Louis DN, Perry A, Wesseling P, et al. The 2021 WHO Classification of Tumors of the Central Nervous System: a summary. Neuro Oncol 2021;23:1231-51. [Crossref] [PubMed]
- Tinkle CL, Campbell K, Han Y, et al. Radiation dose response of neurologic symptoms during conformal radiotherapy for diffuse intrinsic pontine glioma. J Neurooncol 2020;147:195-203. [Crossref] [PubMed]
- Janssens GO, Jansen MH, Lauwers SJ, et al. Hypofractionation vs conventional radiation therapy for newly diagnosed diffuse intrinsic pontine glioma: a matched-cohort analysis. Int J Radiat Oncol Biol Phys 2013;85:315-20. [Crossref] [PubMed]
- Zaghloul MS, Nasr A, Tolba M, et al. Hypofractionated Radiation Therapy For Diffuse Intrinsic Pontine Glioma: A Noninferiority Randomized Study Including 253 Children. Int J Radiat Oncol Biol Phys 2022;113:360-8. [Crossref] [PubMed]
- Zaghloul MS, Eldebawy E, Ahmed S, et al. Hypofractionated conformal radiotherapy for pediatric diffuse intrinsic pontine glioma (DIPG): a randomized controlled trial. Radiother Oncol 2014;111:35-40. [Crossref] [PubMed]
- Tsang DS, Laperriere NJ. Re-irradiation for Paediatric Tumours. Clin Oncol (R Coll Radiol) 2019;31:191-8. [Crossref] [PubMed]
- Perwein T, Giese B, Nussbaumer G, et al. How I treat recurrent pediatric high-grade glioma (pHGG): a Europe-wide survey study. J Neurooncol 2023;161:525-38. [Crossref] [PubMed]
- Massimino M, Vennarini S, Barretta F, et al. How ten-years of reirradiation for paediatric high-grade glioma may shed light on first line treatment. J Neurooncol 2022;159:437-45. [Crossref] [PubMed]
- Tsang DS, Oliveira C, Bouffet E, et al. Repeat irradiation for children with supratentorial high-grade glioma. Pediatr Blood Cancer 2019;66:e27881. [Crossref] [PubMed]
- Kline C, Felton E, Allen IE, et al. Survival outcomes in pediatric recurrent high-grade glioma: results of a 20-year systematic review and meta-analysis. J Neurooncol 2018;137:103-10. [Crossref] [PubMed]
- Amsbaugh MJ, Mahajan A, Thall PF, et al. A Phase 1/2 Trial of Reirradiation for Diffuse Intrinsic Pontine Glioma. Int J Radiat Oncol Biol Phys 2019;104:144-8. [Crossref] [PubMed]
- Lassaletta A, Strother D, Laperriere N, et al. Reirradiation in patients with diffuse intrinsic pontine gliomas: The Canadian experience. Pediatr Blood Cancer 2018;65:e26988. [Crossref] [PubMed]
- Cacciotti C, Liu KX, Haas-Kogan DA, et al. Reirradiation practices for children with diffuse intrinsic pontine glioma. Neurooncol Pract 2020;8:68-74. [Crossref] [PubMed]
- Casey DL, Kushner BH, Cheung NK, et al. Local Control With 21-Gy Radiation Therapy for High-Risk Neuroblastoma. Int J Radiat Oncol Biol Phys 2016;96:393-400. [Crossref] [PubMed]
- Casey DL, Pitter KL, Kushner BH, et al. Radiation Therapy to Sites of Metastatic Disease as Part of Consolidation in High-Risk Neuroblastoma: Can Long-term Control Be Achieved? Int J Radiat Oncol Biol Phys 2018;100:1204-9. [Crossref] [PubMed]
- Liu KX, Naranjo A, Zhang FF, et al. Prospective Evaluation of Radiation Dose Escalation in Patients With High-Risk Neuroblastoma and Gross Residual Disease After Surgery: A Report From the Children's Oncology Group ANBL0532 Study. J Clin Oncol 2020;38:2741-52. [Crossref] [PubMed]
- Gatcombe HG, Marcus RB Jr, Katzenstein HM, et al. Excellent local control from radiation therapy for high-risk neuroblastoma. Int J Radiat Oncol Biol Phys 2009;74:1549-54. [Crossref] [PubMed]
- Casey DL, Kushner BH, Cheung NV, et al. Reduced-Dose Radiation Therapy to the Primary Site is Effective for High-Risk Neuroblastoma: Results From a Prospective Trial. Int J Radiat Oncol Biol Phys 2019;104:409-14. [Crossref] [PubMed]
- Caussa L, Hijal T, Michon J, et al. Role of palliative radiotherapy in the management of metastatic pediatric neuroblastoma: a retrospective single-institution study. Int J Radiat Oncol Biol Phys 2011;79:214-9. [Crossref] [PubMed]
- Lazarev S, Kushner BH, Wolden SL. Short Hypofractionated Radiation Therapy in Palliation of Pediatric Malignancies: Outcomes and Toxicities. Int J Radiat Oncol Biol Phys 2018;102:1457-64. [Crossref] [PubMed]
- Lombardi F, Gandola L, Fossati-Bellani F, et al. Hypofractionated accelerated radiotherapy in osteogenic sarcoma. Int J Radiat Oncol Biol Phys 1992;24:761-5. [Crossref] [PubMed]
- Chen EL, Yoo CH, Gutkin PM, et al. Outcomes for pediatric patients with osteosarcoma treated with palliative radiotherapy. Pediatr Blood Cancer 2020;67:e27967. [Crossref] [PubMed]
- Gaspar N, Hawkins DS, Dirksen U, et al. Ewing Sarcoma: Current Management and Future Approaches Through Collaboration. J Clin Oncol 2015;33:3036-46. [Crossref] [PubMed]
- Haeusler J, Ranft A, Boelling T, et al. The value of local treatment in patients with primary, disseminated, multifocal Ewing sarcoma (PDMES). Cancer 2010;116:443-50. [Crossref] [PubMed]
- Karimi AM, Campbell SR, Parsai S, et al. Aggressive Local Control With Multisite Stereotactic Body Radiation in Metastatic Ewing Sarcoma: A Literature Review and Case Report. Anticancer Res 2020;40:951-5. [Crossref] [PubMed]
- Hayes FA, Thompson EI, Hvizdala E, et al. Chemotherapy as an alternative to laminectomy and radiation in the management of epidural tumor. J Pediatr 1984;104:221-4. [Crossref] [PubMed]
- Koontz BF, Clough RW, Halperin EC. Palliative radiation therapy for metastatic Ewing sarcoma. Cancer 2006;106:1790-3. [Crossref] [PubMed]
- Oberlin O, Rey A, Lyden E, et al. Prognostic factors in metastatic rhabdomyosarcomas: results of a pooled analysis from United States and European cooperative groups. J Clin Oncol 2008;26:2384-9. [Crossref] [PubMed]
- Skamene S, Abish S, Mitchell D, et al. Radiotherapy is Important for Local Control at Primary and Metastatic Sites in Pediatric Rhabdomyosarcoma. Cureus 2015;7:e388. [Crossref] [PubMed]
- Parsai S, Sedor G, Smile TD, et al. Multiple Site SBRT in Pediatric, Adolescent, and Young Adult Patients With Recurrent and/or Metastatic Sarcoma. Am J Clin Oncol 2021;44:126-30. [Crossref] [PubMed]
- Stachelek GC, Terezakis SA, Ermoian R. Palliative radiation oncology in pediatric patients. Ann Palliat Med 2019;8:285-92. [Crossref] [PubMed]
- Khan L, Chiang A, Zhang L, et al. Prophylactic dexamethasone effectively reduces the incidence of pain flare following spine stereotactic body radiotherapy (SBRT): a prospective observational study. Support Care Cancer 2015;23:2937-43. [Crossref] [PubMed]
- Chow E, Meyer RM, Ding K, et al. Dexamethasone in the prophylaxis of radiation-induced pain flare after palliative radiotherapy for bone metastases: a double-blind, randomised placebo-controlled, phase 3 trial. Lancet Oncol 2015;16:1463-72. [Crossref] [PubMed]
- Valdimarsdóttir U, Kreicbergs U, Hauksdóttir A, et al. Parents' intellectual and emotional awareness of their child's impending death to cancer: a population-based long-term follow-up study. Lancet Oncol 2007;8:706-14. [Crossref] [PubMed]
- Mack JW, Wolfe J, Cook EF, et al. Hope and prognostic disclosure. J Clin Oncol 2007;25:5636-42. [Crossref] [PubMed]
- Mack JW, Cronin AM, Kang TI. Decisional Regret Among Parents of Children With Cancer. J Clin Oncol 2016;34:4023-9. [Crossref] [PubMed]
- Mack JW, Fasciano KM, Block SD. Communication About Prognosis With Adolescent and Young Adult Patients With Cancer: Information Needs, Prognostic Awareness, and Outcomes of Disclosure. J Clin Oncol 2018;36:1861-7. [Crossref] [PubMed]
- Mack JW, Wolfe J, Cook EF, et al. Peace of mind and sense of purpose as core existential issues among parents of children with cancer. Arch Pediatr Adolesc Med 2009;163:519-24. [Crossref] [PubMed]
- Rosenberg A, Arnold RM, Schenker Y. Holding Hope for Patients With Serious Illness. JAMA 2021;326:1259-60. [Crossref] [PubMed]
- Mack JW, Wolfe J, Grier HE, et al. Communication about prognosis between parents and physicians of children with cancer: parent preferences and the impact of prognostic information. J Clin Oncol 2006;24:5265-70. [Crossref] [PubMed]
- Hilden JM, Emanuel EJ, Fairclough DL, et al. Attitudes and practices among pediatric oncologists regarding end-of-life care: results of the 1998 American Society of Clinical Oncology survey. J Clin Oncol 2001;19:205-12. [Crossref] [PubMed]
- Sisk BA, Kang TI, Mack JW. Prognostic disclosures over time: Parental preferences and physician practices. Cancer 2017;123:4031-8. [Crossref] [PubMed]
- Baile WF, Lenzi R, Parker PA, et al. Oncologists' attitudes toward and practices in giving bad news: an exploratory study. J Clin Oncol 2002;20:2189-96. [Crossref] [PubMed]
- Bluebond-Langner M, Belasco JB, Goldman A, et al. Understanding parents' approaches to care and treatment of children with cancer when standard therapy has failed. J Clin Oncol 2007;25:2414-9. [Crossref] [PubMed]
- Mack JW, Joffe S, Hilden JM, et al. Parents' views of cancer-directed therapy for children with no realistic chance for cure. J Clin Oncol 2008;26:4759-64. [Crossref] [PubMed]
- Mack JW, Feudtner C, Hinds PS. Communication and decision support for children with advanced cancer and their families. Am Soc Clin Oncol Educ Book 2012;637-43. [Crossref] [PubMed]
- Rosenberg AR, Wolfe J, Wiener L, et al. Ethics, Emotions, and the Skills of Talking About Progressing Disease With Terminally Ill Adolescents: A Review. JAMA Pediatr 2016;170:1216-23. [Crossref] [PubMed]
- Kaye EC, Rockwell S, Woods C, et al. Facilitators Associated With Building and Sustaining Therapeutic Alliance in Advanced Pediatric Cancer. JAMA Netw Open 2021;4:e2120925. [Crossref] [PubMed]
- October TW, Dizon ZB, Arnold RM, et al. Characteristics of Physician Empathetic Statements During Pediatric Intensive Care Conferences With Family Members: A Qualitative Study. JAMA Netw Open 2018;1:e180351. [Crossref] [PubMed]